In the recently published ‘Three-dimensional printing in structural heart disease and intervention,’ authors Yiting Fan, Randolph H.L. Wong, and Alex Pui-Wai Lee, all from The Chinese University of Hong Kong, explore the potential for 3D printing in the world of medicine, as well as cardiology—and more specifically, structural heart disease (SHD).
SHD causes issues like:
- Aortic stenosis
- Mitral regurgitation
- Atrial septal defect
- Left atrial appendage (LAA) clots
Conventional imaging is limited, while the emergence of 3D printed models allows medical professionals to progress from mentally reconstructing 2D images to gaining a more complex understanding of pathology.
As 3D printing continues to make its way into the realm of medicine, models are used for:
- Guiding treatment
- Procedural simulation
- Facilitating hemodynamic research
- Improving interventional training
- Promoting patient-clinician communication
To create a medical model, images must be attained, data must be processed, and the object must be 3D printed.
“The most commonly used imaging sources for SHD are echocardiography, computed tomography (CT) and magnetic resonance imaging (MRI),” state the researchers. “Other modalities, such as positron emission tomography, single photon emission CT and cone beam CT, are less commonly used. All images should use the common Digital Imaging and Communication in Medicine (DICOM) format.
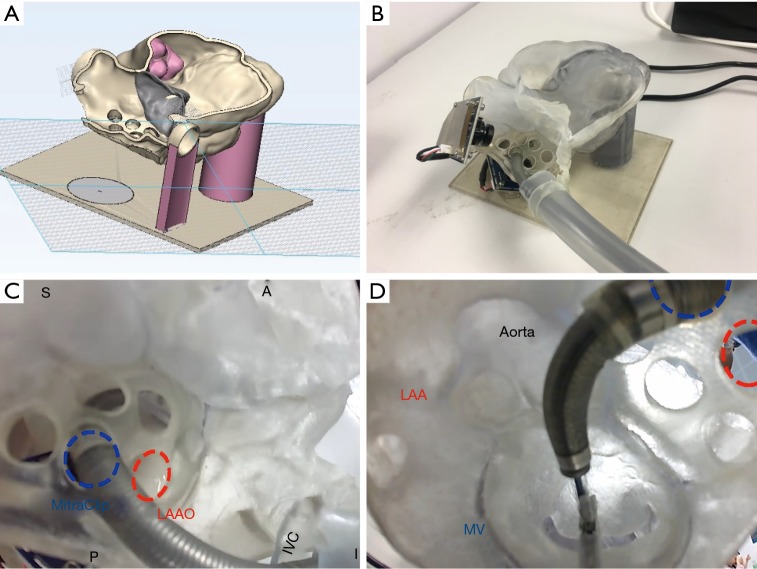
Pre-procedural simulation of MitraClip on 3D-printed model. (A) Digital model of a heart. Different colors stand for different cardiac components (grey: mitral valves; light pink: tricuspid valves; light gold: atrial septum, left atrium, left and right ventricle). (B) Multi-material 3D-printed heart model for pre-procedural simulation. The valves were printed with flexible material and the rests were printed with hard material. (C) The 5 holes drilled in the atrial septum represents the different position for different kinds of structural heart interventions. (D) The MiraClip device was released via delivery catheter through the atrial septum to the mitral valve. Blue circle: MitraClip; red circle: left atrial appendage occlusion (LAAO). S, superior; A, anterior; P, posterior; I, inferior; IVC, inferior vena cava; LAA, left atrial appendage; MV, mitral valve.
A range of materials are both popular and possible for fabricating medical models:
“Multi-material printing by material jetting is increasingly used to create cardiac structures. Different tissue components were printed with different textures. For instance, an aortic valve was printed with flexible printing material, and the calcifications attached to valves were printed with hard printing material, respectively,” report the authors.
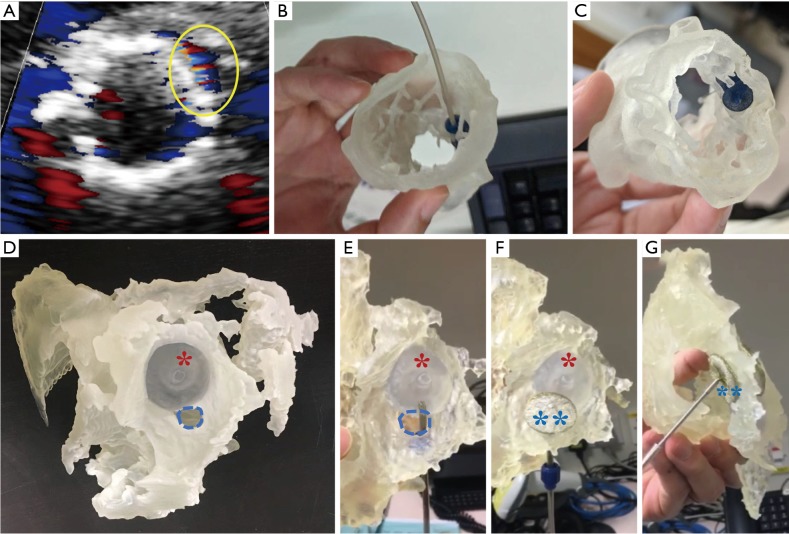
Application of 3D printing for peri-device leak. (A,B,C) A case found with peri-device leak post TAVI and needed peri-device leak occlusion: (A) routine TEE post-TAVI showed peri-device leak (yellow circle); (B) simulation of peri-device leak occlusion on 3D-printed aortic root model derived from post-TAVI CT; (C) the 10-mm vascular plug was found to be best-fit for this case. (D,E,F,G) A case found with residual leak after ASD closure: (D) multi-material 3D printed model showed residual leak (blue circle) next to the ASD occluder (asterisk *); (E) the delivery catheter went through the leak position; (F) the device (two asterisks **) was released in situ. (G) The bicaval view of 3D-printed model showed stable release and stay of the chosen device. 3D, three-dimensional; TAVI, transcatheter aortic valve implantation; TEE, transesophageal echo; CT, computed tomography; ASD, atrial septal defect.
There continue to be ongoing challenges in the creation of medical models, however, ‘despite the enthusiasm in applying 3D printing cardiovascular medicine.’ While there is an obvious lack of technical standards, mainly due to the novelty of the technology, the authors point out also that there are still issues with affordability—along with ‘scant evidence on the added clinical benefit.’
Greater accuracy is needed, along with improved standardization of data acquisition, and post-processing techniques. While deeper research is required into the creation and use of models and surgical guides, so are comparisons for offering up better information and creating industry standards. The authors also recommended a more streamlined workflow.
“The mechanical properties of the 3D-printed materials, such as tensile strength, elasticity, flexibility, hardness, and durability have utmost importance for cardiovascular applications. The majority of cardiovascular applications reported so far have employed materials with properties that have not been meticulously compared with the cardiovascular tissue they are mimicking. Validation of 3D-printed material properties against actual human patient tissues is important to ensure that procedural simulation is realistic,” conclude the authors.
“Further effort in technical standardization, and clinical evaluation of added benefit and cost-effectiveness of 3D printing are needed to bring this promising technique to clinical reality.”
3D printed medical models are extremely beneficial to doctors and patients as they allow not only for diagnosing but have also continued to change medicine—allowing for procedures involving complex reconstructions, fabrication of surgical guides, and much more.
What do you think of this news? Let us know your thoughts! Join the discussion of this and other 3D printing topics at 3DPrintBoard.com.
[Source / Images: ‘Three-dimensional printing in structural heart disease and intervention’]
The post Chinese University of Hong Kong Studies 3D Printing for Heart Disease appeared first on 3DPrint.com | The Voice of 3D Printing / Additive Manufacturing.