According to the World Health Organization, cardiovascular disease is the number one cause of death globally. An estimated 17.9 million people died from cardiovascular disease in 2016, representing 31% of all global deaths. Treatments for all manner of cardiovascular impairments are therefore a high priority. A very prevalent ailment in that category is a heart attack which happens to over 735,000 Americans a year.
A team of researchers led by Dr. Vahid Serpooshan, an assistant professor of Biomedical Engineering and Pediatrics at Georgia Institute of Technology and Emory University School of Medicine, have created a patch with a regenerative protein to treat the infarcted myocardium (cardiac muscle, a part of the heart that gets damaged during a heart attack). According to Georgia Institute of Technology the patch will cover the infarcted tissue with a collagen patch infused with a protein called FSTL1, which is tailored to fit an area a little larger than the dead tissue. Dr. Serpooshan used bioprinters to develop the patch using a collagen gel. The patch is about to start its pre-clinical trial phase at Emory University’s School of Medicine in Atlanta, and a clinical trial is already ongoing in Europe for a version of this patch device. So it could be a couple more years before they are available for patient treatment. Dr. Serpooshan’s Lab (which has two locations at both Emory University and Georgia Tech) uses a multidisciplinary approach to design and develop micro/nano-scale tissue engineering technologies with the ultimate goal of generating functional tissues and organs. 3DPrint.com reached out to talk to Dr. Serpooshan about his team’s groundbreaking work.
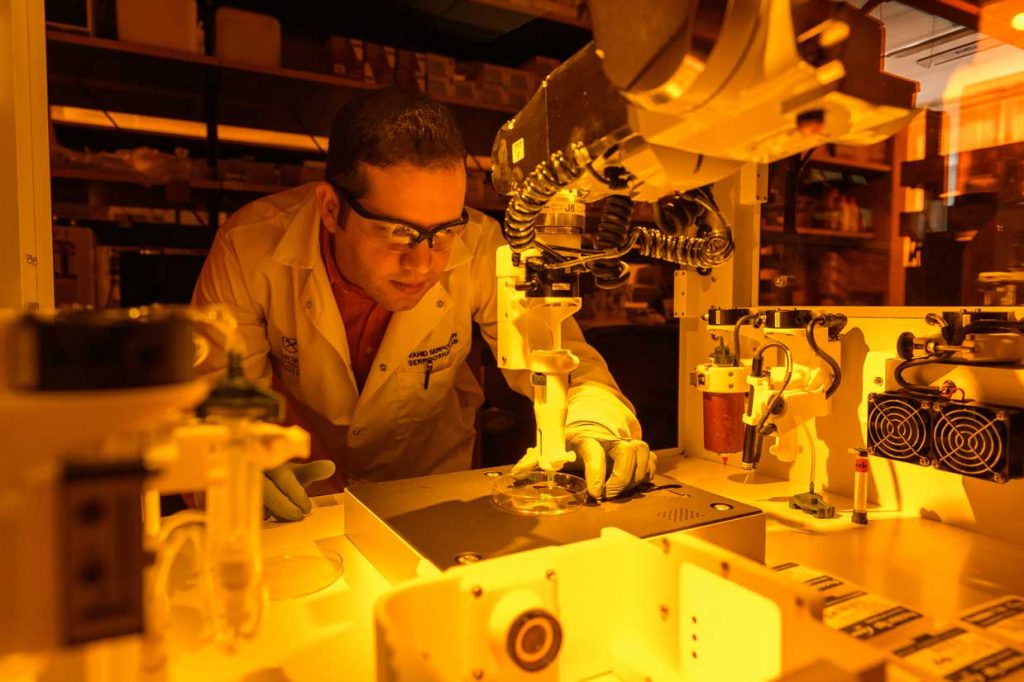
Professor Vahid Serpooshan sets up the medical 3D printer that will print a patch engineered to strengthen heart muscle damaged in a heart attack. (Photo by Rob Felt, Georgia Institute of Technology)
How has 3D printing helped to engineer the patch?
3D printing has been a huge help in manufacturing the patch in so many ways. It enabled us to incorporate vasculature inside the patch, something that would not have been available with traditional tissue engineering techniques, since they offer really limited potential to create complex, functional vasculature in thick, 3D tissue constructs. Additionally, it allowed us to have a controlled deposition of different cells, biomaterials, and molecules to form heterogenous, complex 3D structures that closely mimic native tissue structure. Also it provided us with patient -and disease- specificity: we utilize MR or CT imaging data obtained from patients to create 3D printed tissue constructs that precisely match the patient’s diseased or damaged tissue. Finally, bioprinting technologies have enabled tissue engineers to markedly enhance resolution and precision in various tissue manufacturing endeavors, making precision medicine even more promising.
What 3D printer do you use to create the patch?
We are mainly using two types of 3D bioprinters for this project. A BioAssemblyBot bioprinting system, made by Advanced Solutions Inc. (an American company in Louisville, Kentucky). This is the ONLY six-axis robotic arm printer in the market, with a resolution down to approximately 20 to 50 μm (microns). The second bioprinter is a BIO-X from Swedish biotech company Cellink, a pioneer in bioprinting.
How long will it take to manufacture the patch?
Biomanufacturing of a cardiac patch at a clinically-relevant scale would take about 15 to 30 minutes, depending on the number of cell types involved, the vasculature design, and other factors. This enables the clinics, hopefully in the near future, to have heart attack patients come to the clinic, conduct CT or MR imaging, prepare 3D digital model of the damaged tissue, and sending it to the bioprinter to manufacture a patch device customized for that patient.
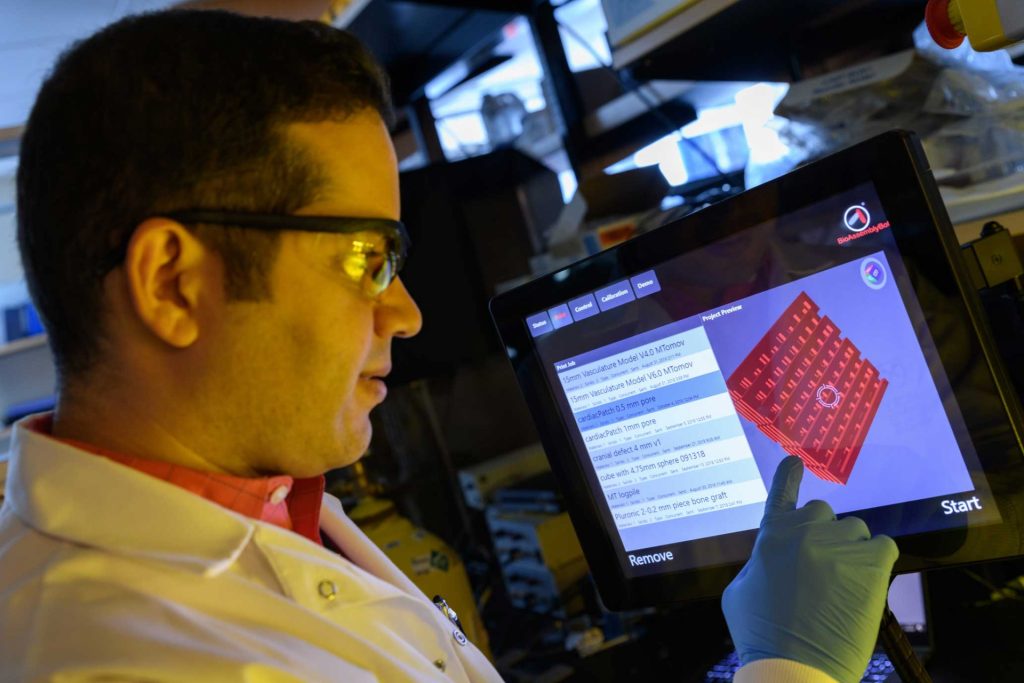
Vahid Serpooshan designing the myocardial infarct patch (Photo by Rob Felt, Georgia Institute of Technology)
Is there already a procedure in place to apply the patch?
Actually, there already is a procedure to apply the patch. We use a left thoracotomy approach to get access to the surface of the heart and suture the patch onto the epicardial layer of the heart, covering the myocardial infarction (also called a heart attack) area. There are currently some ongoing works trying to minimize the aggressiveness of this surgical procedure, potentially using catheter-based techniques.
What are the benefits of using collagen in the patch?
Collagen is the most abundant protein of the human body. This protein highly supports cell viability, proliferation, and function. It is a biodegradable protein which is a major advantage for in vivo applications, facilitating timely integration of the implant with the host tissue and avoiding long-term immunogenic complications. For our 3D bioprinting works, we use gelatin methacrylate (gelMA). Gelatin is derived from collagen (a hydrolyzed collagen) and offers similar advantages. The main advantages of gelMA for bioprinting of cardiac patches include: acceptable compatibility with cardiac cells (cardiomyocytes), tunable biomimetic mechanical properties (stiffness), controllable degradation rate, and of course, great printability (rheology properties).
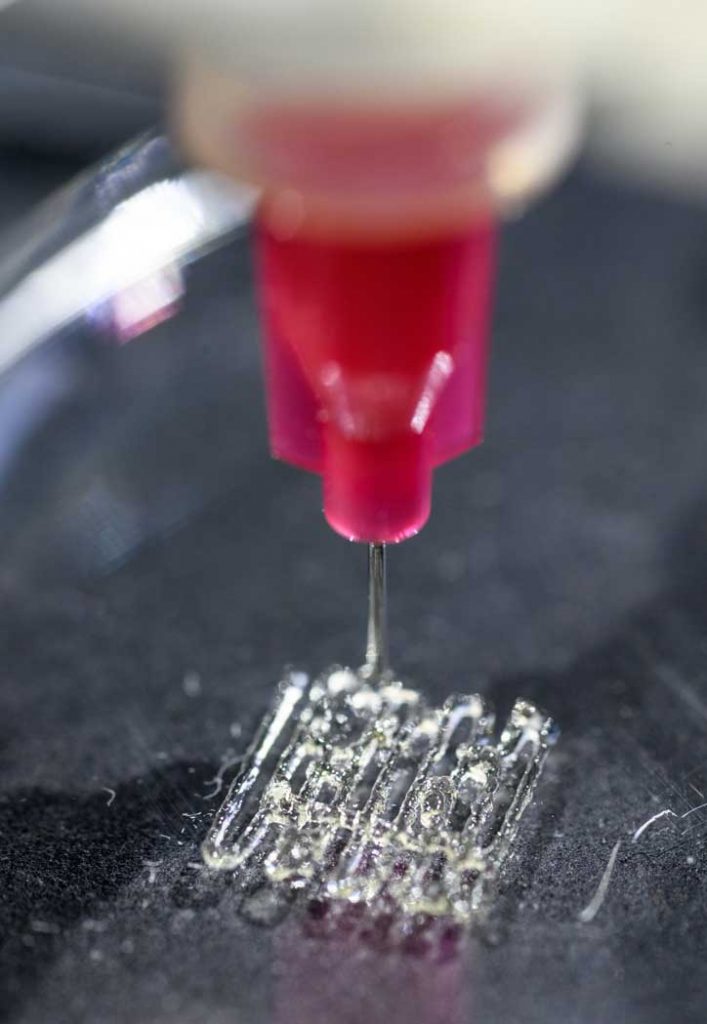
The collagen gel used to develop the 3D printed patch. (Photo by Rob Felt, Georgia Institute of Technology)
What percentage of heart disease patients will benefit from this device?
This therapy is designed for a specific group of heart attack patients, approximately one third of patients who either arrive too late to the clinics or are resistant to current catheter-based reperfusion methods and pharmacologic therapies.
If approved, Dr. Serpooshan’s patch could help over 200,000 patients per year.