Japanese researchers Jin Liu, Tatsuaki Tagami, and Tetsuya Ozeki have completed a recent study in nanomedicine, releasing their findings in “Fabrication of 3D Printed Fish-Gelatin-Based Polymer Hydrogel Patches for Local Delivery of PEGylated Liposomal Doxorubicin.” Experimenting with a new drug delivery system, the authors report on new potential for patient-specific cancer treatment.
The study of materials science continues to expand in a wide range of applications; however, bioprinting is one of the most exciting techniques as tissue engineering is expected to lead to the fabrication of human organs in the next decade or so. Such research has also proven that bioprinting may yield much more powerful drug delivery whether in using hybrid systems, multi-drug delivery systems, or improved scaffolds.
Here, the materials chosen for drug delivery are more unique as the researchers combined printer ink with semi-synthesized fish gelatin methacryloyl (F-GelMA)—a cold fish gelatin derivative.
In providing aggressive cancer treatment to patients, the use of doxorubicin (DOX) is common as an anti-carcinogen for the treatment of the following diseases:
- Breast cancer
- Bladder cancer
- Kaposi’s sarcoma
- Lymphoma
- Acute lymphocytic leukemia
DOX may also cause serious cardiotoxicity, however, despite its use as a broad-spectrum drug. As a solution, PEGylated liposomal DOX, Doxil has been in use for treatment of cancer with much lower cardiotoxity. The nanomedicine has also been approved by the FDA, and is used for targeting local tumors; for instance, this type of drug delivery system could be suitable for treating a brain tumor.
“PEGylating liposomes can prolong their circulation time in blood, resulting in their passive accumulation in cancer tissue, called the enhanced permeability and retention effect,” state the authors.
Using a 3D bioprinter, the authors developed liposomal patches to be directly implanted into cancerous cells.
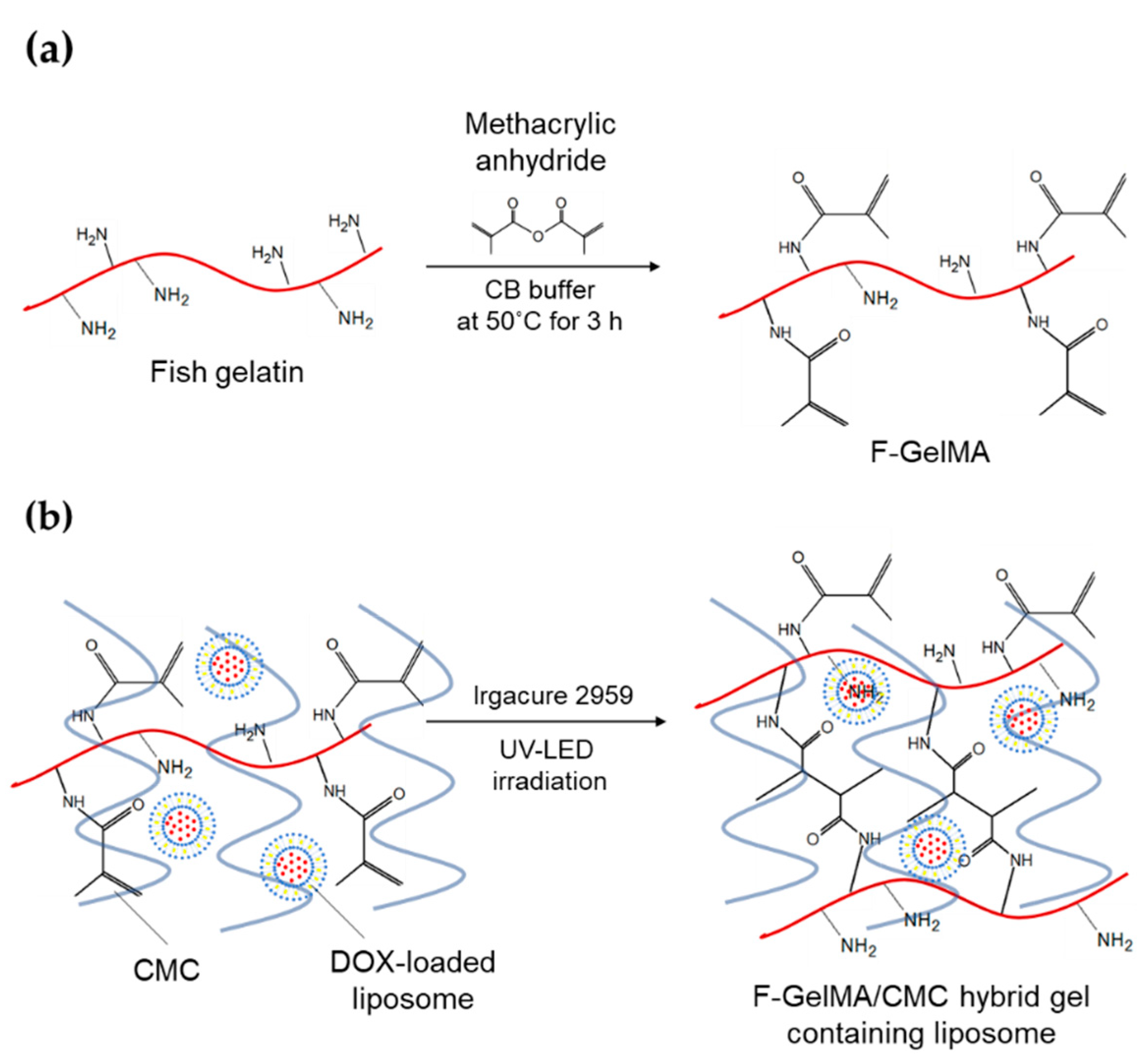
(a) Synthesis of fish gelatin methacryloyl (F-GelMA). (b) Hybrid gel of cross-linked F-GelMA and carboxymethyl cellulose sodium (CMC) containing PEGylated liposome. The reaction scheme was prepared in previous studies
“We used a hydrogel containing semi-synthetic fish-gelatin polymer (fish gelatin methacryloyl, F-GelMA) to entrap DOX-loaded PEGylated liposomes. Fish gelatin is inexpensive and faces few personal or religious restrictions,” stated the authors.
Fish gelatin has not been used widely in bioprinting, however, due to low viscosity and rapid polymerization. To solve that problem, the authors created a bioink composite with elevated viscosity.

Viscous properties of drug formulations used as printer inks. (a) The appearance of F-GelMA hydrogels containing different concentrations of CMC. (b) The viscosity profiles of F-GelMA hydrogels containing different concentrations of CMC. The data represent the mean ± SD (n = 3).
And while hydrogels are generally attractive for use due to their ability to swell, for this study, the researchers fabricated a variety of different materials—with the combination of 10% F-GelMA and 7% carboxymethyl cellulose sodium (a thickening agent) showing the highest swelling ratio.
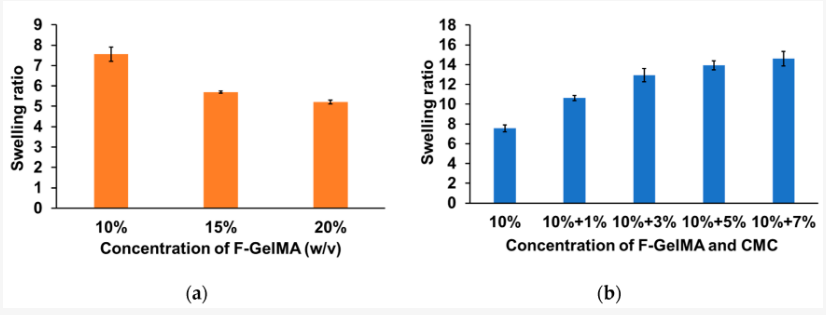
Swelling properties of hydrogels after photopolymerization. (a) Swelling ratio of different concentrations of F-GelMA. (b) Swelling ratio of mixed hydrogel (10% F-GelMA with different concentrations of CMC). The data represent the mean ± SD (n = 3).
Patches were printed in three different sample shapes, using a CELLINK bioprinter syringe as the authors tested drug release potential in vivo. Realizing that surface area, crosslinks density, temperature, and shaker speed would play a role, the team relied on a larger surface volume for more rapid release of drugs.
While experimenting with the torus, gridline, and cylindrical sample patches, the researchers observed gridline-style patches as offering the greatest potential for sustained release.

Drug release profiles of liposomal doxorubicin (DOX). (a) Influence of shape on drug release. The UV exposure time was set to 1 min. (b) Influence of UV exposure time on drug release. The gridline object was used for this experiment. The data represent the mean ± SD (n = 3).
“These results indicate that CMC is useful for adjusting the properties of printer ink and is a useful and safe pharmaceutical excipient in drug formulations. We also showed that drug release from 3D-printed patches was dependent on the patch shapes and UV exposure time, and that drug release can be controlled. Taken together, the present results provide useful information for the preparation of 3D printed objects containing liposomes and other nanoparticle-based nanomedicines,” concluded the authors.
[Source / Images: ‘Fabrication of 3D Printed Fish-Gelatin-Based Polymer Hydrogel Patches for Local Delivery of PEGylated Liposomal Doxorubicin’]
The post 3D Printed Medicine Uses Fish Gelatin to Deliver Cancer Treatment appeared first on 3DPrint.com | The Voice of 3D Printing / Additive Manufacturing.