Researchers are looking into ways to optimize biomedical monitoring, with their results outlined in ‘A 3D Printed High-Dielectric Filled Elliptical Double-Ridged Horn Antenna for Biomedical Monitoring Applications.’ Seeking to make further impacts in the field of medical science, the team from Queen Mary University of London has 3D printed an innovative device for sensing applications with wireless technology—based on ultra-wideband devices initially created for short-range wireless communications.
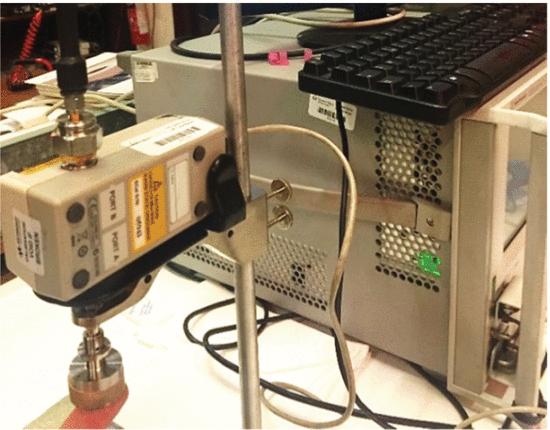
The in-house measurement setup based on the open-ended coaxial probe technique, used for the characterization of the dielectric materials.
Created to work within UK Communications Industries (Ofcom) and the Federal Communications Commission (FCC) regulation of UWBs, the new device has been found to offer depth suitable for penetration in scanning skin, muscle, and fat, with signals able to sense layer thicknesses. Wide-band technologies are often used for short-range communication due to:
- Low power
- High data rates
- Multipath immunity
- Simultaneous ranging and communication
While this type of antenna is not new, the use of 3D printing is novel. The double-ridged horn has been a topic of research over the years for researchers because of the benefits, leading to a more effective answer to refining accuracy in biomedical scanning. And while 3D printing can offer greater affordability in many cases, here the research team was concerned about cost-prohibitive fabrication, so they compared materials, ultimately settling on in-house 3D printing with ABS.
The shape of the horn allows for better operation overall, and the high dielectric material allows for a miniaturized design that also reduces reflection and is both easy and affordable to make. With an extension, the scientists were able to expand on the antenna and prevent signal-overlapping issues.

Modeled extended EDRH antenna with the structural labels and the dimensions for the extended section.
“The optimal approach is to extend the outer aperture of the antenna, and to define, the antenna outer aperture length, so the scanning tissue area can be placed in the far-field region,” stated the researchers. “This has added more complexity to the fabrication and realization of the device with the increased cost, but on the other hand, it has made it more stable in its operation, and free of any destructive interference signals and noise.”
The team used the Stratasys Objet30 Prime 3D printer for creating their prototype, finishing it with clear Vero polyethylene, stating that hardware and materials not only offered high resolution, accuracy, and conductivity, but also affordability in fabrication.
Measurements were found to be accurate also, as they addressed concerns regarding individual and other influences like scanning areas and layer structure but concluded that there should be very little variance between ‘permittivity and thickness.’ If an impact on the results was noted, the researchers explained that added calibration measures could be taken with an open-ended probe, with software producing the results.

(a) 3D-printed EDRH antenna using the polyethylene material. (b) 3D-printed EDRH antenna, as conductive-painted and fed with a semi-ridged SMA connector. (c) 3D-printed EDRH antenna filled with the high-dielectric mixture.
“This design incorporates the extension for locating the antenna in the far-field region of the scanning area, for the plane-waves to penetrate more directly into the body. Moreover, the antenna can operate at the lower frequency band of WB to exhibit a better penetration depth and impedance matching using the mixture for the biomedical application, which monitoring very deep inside the body is the main objective of the system,” concluded the researchers at the end of their study.
3D printing has offered much greater expansion opportunities for scientists and engineers interested in creating better devices for sensing and monitoring, from automotive sensors to electrochemical sensing, and 3D printed models for better monitoring.
What do you think of this news? Let us know your thoughts! Join the discussion of this and other 3D printing topics at 3DPrintBoard.com.
[Source / Images: ‘A 3D Printed High-Dielectric Filled Elliptical Double-Ridged Horn Antenna for Biomedical Monitoring Applications’]
The post London: 3D Printing the Double-Ridged Horn Antenna for Biomedical Monitoring appeared first on 3DPrint.com | The Voice of 3D Printing / Additive Manufacturing.