In order to tailor and improve the performance of microstructures, it helps with many 3D-printed alloys if the post-heat treatment process is carefully designed and executed for this purpose. Researchers Yunhao Zhao, Noah Sargent, Kun Li, and Wei Xiong with the University of Pittsburgh’s Physical Metallurgy and Materials Design Laboratory published a paper, “A new high-throughput method using additive manufacturing for materials design and processing optimization,” about their work on this subject, which was supported by a NASA contract.
They explained that post-heat treatment optimization and composite design are the central parts of materials development, and that “high-throughput (HT) modeling and experimentation are critical to design efficiency.” These aspects are even more important when it comes 3D printing, because the more processing parameters are used, the more the “microstructure-property relationships of the as-fabricated materials” will be effected.
“In this work, we couple the [laser powder bed fusion (LPBF) technique with the gradient temperature heat treatment (GTHT) process as an effective HT tool to accelerate the post-heat treatment design for AM components,” they explained.
They used the Ni-based Inconel 718 superalloy, which has excellent high-temperature mechanical properties, in order to evaluate their proof of concept, as the material is often fabricated with LPBF technology.
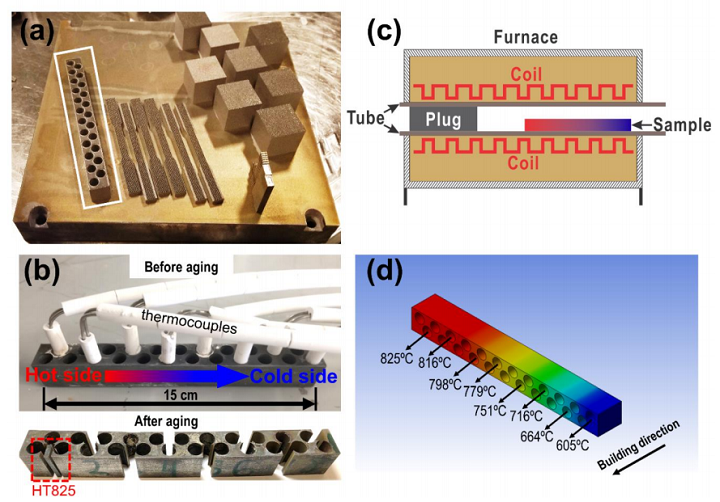
Figure 1. (a) Inconel 718 build printed by LPBF; (b) setup of temperature record and illustration of sample cutting for microstructure characterization; (c) setup of the furnace for the high-throughput experiment; (d) experimental temperature distribution inside the bar-sample.
The researchers created a high-throughput approach by using LPBF technology to print a cuboid long-bar sample out of Inconel 718 on an EOS M290. They designed the build with 23 evenly distributed holes, which not only increase the sample’s surface area and improve convection heat transfer, but also make it more flexible “when choosing monitoring locations.” The improved heat transfer also helped lower the variation in the sample’s temperature relative to the temperature of the air.
“As a result, the air temperature calibration became more representative of the real sample temperature, which allowed the preemptive selection of the monitoring locations in the sample according to the actual needs. Using this methodology, the current work significantly reduced the total time needed for heat treatment, and the flexibility of the setup of the high-throughput experiment was increased by adopting additive manufacturing methods for sample fabrication,” they explained.
Once the long bar sample’s microsegration and AM-related grain texture had been removed, it was submerged in ice water, and then conductive high-temperature cement was used to fix eight K-type thermocouples into equidistant holes. Finally, it was time for the 15-hour aging process of the heat treatment.
“The thermocouples were connected to a computer via a data acquisition system to record the aging temperatures at each location throughout the aging process,” the researchers wrote. “The aging heat treatment was then carried out in a tube furnace with one end open to introduce gradient temperatures at different locations in the sample, as illustrated in Fig. 1(c). The furnace temperature settings and the position of the sample inside of the furnace tube had been deliberately calibrated to acquire a temperature gradient of 600~800°C, within which the δ, γ′, and γ″ phases may precipitate during the aging processes [19]. The temperature gradient during the aging process is stable without fluctuation, and the distribution of temperatures achieved at each monitored location is illustrated in Fig. 1(d). From Fig. 1(d), the experimentally obtained temperature gradient was within 605~825°C, which agreed well with our expectation.”
The adjacent alloy to each thermocouple was individually sectioned to characterize the microstructure, and view the effect of the various aging temperatures. After the samples were polished, they were analyzed with SEM (scanning electron microscope), so the team could identify the phases, and EBSD (electron backscatter diffraction), for grain morphology observation.
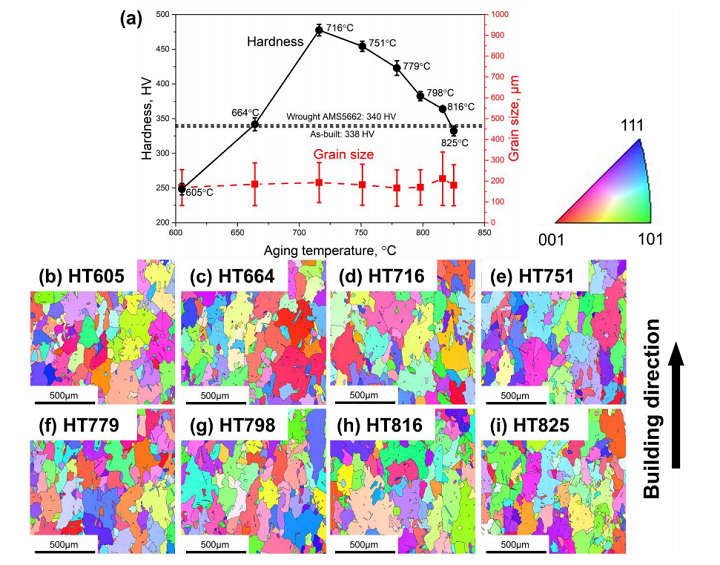
Figure 3. (a) Results of microhardness and average grain size measurements. IPFs of the aged samples with (b) HT605; (c) HT664; (d) HT716; (e) HT751; (f) HT779; (g) HT798; (h) HT816; (i) HT825.
“Within the temperature range of 716~816°C, the hardness of the aged samples are higher than that in the wrought Inconel 718 (340 HV, AMS5662) [14], indicating the AM alloys could achieve higher strengthening effects when applied suitable heat treatment,” they wrote. “The highest hardness is 477.5 HV0.1 and occurs after aging at a temperature of 716°C. It is found that the temperatures above and below 716°C result in the reduction of hardness. The lowest hardness of 248.4 HV0.1 is obtained at 605°C, which is lower than that in the as-built alloy (338 HV0.1).”
The EBSD found that coarse grains formed in all of the aged samples, and while their diameters were “plotted as a function of the corresponding aging temperatures in Fig. 3(a),” their size is independent of the temperature. This likely means that the aging temperatures did not significantly effect either the grain size or morphology, and that “the relatively large grain size achieved after heat treatment in this study has little contribution to the microhardness variation.”
To better understand structure-property relationships, the researchers chose three samples to undergo more microstructure investigation:
- HT605 with the lowest microhardness of 248.4 HV0.1,
- HT716 with the highest microhardness of 477.5 HV0.1, and
- HT825 with the lowest microhardness of 332.2 HV0.1 in the high-temperature gradient
Other than a few NbC carbides, they did not see any other precipitates in the HT605 sample, but noted that 716°C-aging caused a little “of the δ phase to precipitate along grain boundaries” in the HT716 sample.
“However, a large number of plate-shaped γ″ particles are observed in the TEM micrographs,” the team wrote. “These γ″ particles are very fine with a mean particle length of 13.8±4.2 nm through image analysis. The typical γ′ phase with spherical shape is not found to precipitate in sample HT716. This indicates that the precipitation of γ″ preceded the formation of γ′ in the current study. Therefore, the strengthening effect is dominated by γ″ with fine particle size.”

Figure 4. Microstructures of HT605 characterized by (a) SEM-BSE; (b) bright-field TEM; (c) selected-area-electron-diffraction (SAED). Microstructures of HT716 characterized by (d) SEM-BSE; (e) bright-field TEM; (f) SAED. Microstructures of HT825 characterized by (g) SEM-BSE; (h) bright-field TEM; (i) SAED. The different γ″ variants in (f) and (i) are differently colored, and the corresponding zone axes are indicated.
Just like with the second sample, the researchers also did not observe the γ′ phase in HT825.
The team deduced that the phase transformation behaviors caused the varying microhardnesses in the aged samples, concluding that aging the 3D-printed Inconel 718 samples at 605°C for 15 hours is not ideal for precipitation-hardening.
“We developed a high-throughput approach by fabricating a long-bar sample heat-treated under a monitored gradient temperature zone for phase transformation study to accelerate the post-heat treatment design of AM alloys. This approach has been proven efficient to determine the aging temperature with peak hardness. We observed that the precipitation strengthening is predominant for the studied superalloy by laser powder bed fusion, and the grain size variation is insensitive on temperature between 605 and 825ºC.”
Discuss this and other 3D printing topics at 3DPrintBoard.com or share your thoughts below.
The post Gradient Temperature Heat Treatment of LPBF 3D-Printed Inconel 718 appeared first on 3DPrint.com | The Voice of 3D Printing / Additive Manufacturing.