Just as a New Zealand-based surfer was inspired by the humpback whale and the microgrooves of shark skin when creating his surfboard fins, so too was a team of international researchers inspired by the natural world in their structure study of an on-water sports board. In their recently published paper, “3D Printing On-Water Sports Boards with Bio-Inspired Core Designs,” they explain their work advancing the board by using 3D printing and different bio-inspired core structures, such as the honeycomb.
“Modeling and analyzing the sports equipment for injury prevention, reduction in cost, and performance enhancement have gained considerable attention in the sports engineering community. In this regard, the structure study of on-water sports board (surfboard, kiteboard, and skimboard) is vital due to its close relation with environmental and human health as well as performance and safety of the board,” the researchers wrote.
3D printing has often been used in the sports field, but in previous studies about 3D printed boards, researchers mainly focused on the geometry, only making small modifications to the equipment. This research team actually introduced different patterns to use as the board’s internal core structure. FDM technology and PLA materials were used to make the first sample board, featuring a uniform honeycomb structure that was created with the help of CATIA V5 software.
Most modern boards feature a sandwich structure, where a thin outer shell covers an inner core made of foam, which allows for increased buoyancy and stability, less weight, and improved bending resistance. These structures typically feature a top shell, the lightweight core, and a bottom shell, but this board merged the bottom shell with the core.
“A smaller scale version of a real on-water sports board was designed,” the researchers wrote. “The board had a 48 mm width and 144 mm length with a 357 mm radius curvature at two sides. A bottom curvature of 600 mm was considered, resulting in a model closer to the real one. The hexagonal honeycomb structure formed the core of the board, and was repeated across the specimen.”
The honeycombs were 3 mm wide, and patterned with 1 mm thick walls, while the bottom and top shells had thicknesses of 5 and 1.5 mm, respectively. The team used an XYZprinting da Vinci 1.0 Pro 3D printer to make the sample board with a uniform honeycomb structure.
Surfboard fractures frequently happen between the surfer’s feet, in the board’s middle section. Usually, this occurs because the lip of the wave impacts in the middle and rips it into two parts after the surfer falls into the water, or because the surfer’s feet get too close together and concentrate their body’s pressure in the middle.
“In both of these circumstances, an immense force acts upon the middle portion of the board, causing large bending stress that may result in breakage,” the researchers explained.
“As both of these breakages are caused by bending stresses, a mechanical three-point bending test could be employed to determine the strength of the board in such loading.”
The team tested the honeycomb board under 3-point loading, though they had to change the grippers for the test.
“The test with the strain rate of 0.001 s−1 was carried out at room temperature with an 80 mm distance between two supports. A displacement-controlled test was conducted to get a maximum deflection of 4 mm in the elastic range.”
In order to validate these results, and model the structure’s deformation under the test, the researchers developed a “geometrically linear analytical method,” using an equivalent I-shaped section with geometrical stiffness varied along the X-axis, to simulate the honeycomb structure. Then, a geometrically non-linear finite element method, based on ABAQUS software, simulated the boards with a variety of different core structures under the three-point bending test.
A bending test was simulated to validate the FEM model, and the team performed a mesh sensitivity analysis to make sure the numerical results were accurate. Then, they applied the same test to the sample board with the honeycomb core for a 4 mm maximum deflection. The maximum stress of ∼40 MPa, found in the middle of the board, was low enough to keep the board “in the desired elastic region.” For comparison, the PLA had a yield test level of 60 MPa.
“The force–deflection curve for the experimental, geometrically non-linear numerical, and geometrically linear analytical results are plotted and compared to each other in Figure 13,” the researchers explained. “The preliminary conclusion drawn from this figure is the fact that the PLA board shows a linear elastic deformation up to 300 N force, beyond which the material yields, followed by plastic deformation that is manifested as a plateau after 500 N.”
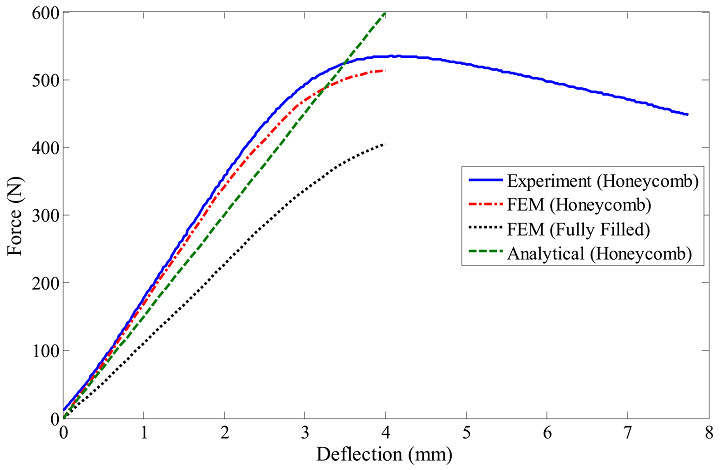
Comparison of the experimental, numerical, and analytical load–deflection curves for the three-point bending test of the honeycomb and fully-filled boards.
Once the team had validated the geometrically non-linear FEM model for the board with the honeycomb core structure, they simulated other patterns for the bottom shell’s core. Performing the three-point bending test with the geometrically non-linear FEM software package ABAQUS, while the board’s total volume was kept constant, helped them find the structure with the maximal bending resistance. The different structures they tested were:
- Hexagonal-Rhomic (HR) Structure
- Triangular Honeycomb Structure
- Hexagonal Carbon Lattice
- Pine Cone and Sunflower-Inspired Patterns
- Spiderweb-Inspired Pattern
- Functionally Graded (FG) Honeycomb Structure
“For all of the structures, the mesh convergence study was conducted and the appropriate number of elements for the FEM model was selected,” the researchers wrote. “Furthermore, the maximum stresses of all boards with various core structures were figured to have shown a maximum stress lower than the yield stress of the PLA material.”
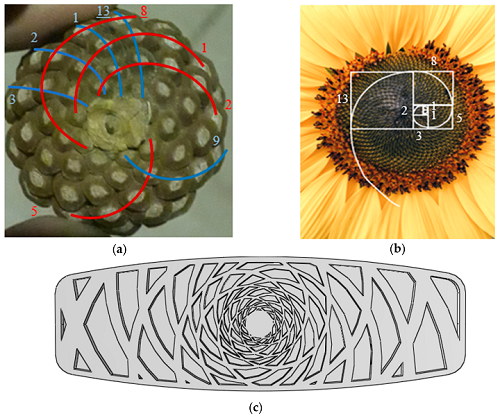
(a) A pinecone with two 8-number and 13-number opposite directional spirals; (b) Sunflower with Fibonacci spiral; (c) Pinecone-inspired structure designed using Fibonacci spirals.
They found that the board with the FG honeycomb structure had the best bending performance – 31% better, in fact, than the board with the uniform honeycomb structure at 500 N force. This means that it can tolerate maximum forces, as opposed to an intermediate force like the rest of the structures.
“Due to the absence of similar designs and results in the literature, this paper is expected to advance the state of the art of on-water sports boards and provide designers with structures that could enhance the performance of sports equipment,” the researchers concluded.
Discuss this and other 3D printing topics at 3DPrintBoard.com or share your thoughts below.
The post 3D Printed Surfboard: Researchers Test Different Bio-Inspired Core Structures appeared first on 3DPrint.com | The Voice of 3D Printing / Additive Manufacturing.