We’re starting out with some formnext news in today’s 3D Printing News Briefs, as the show is currently taking place in Frankfurt this week. SCANLAB is introducing a new scan system control extension at the show. We also have some metal stories today – Desktop Metal has launched 4140 chromoly steel for its Studio System, while QuesTek Innovations and the German Aerospace Center are exploring the potential of a high-temperature aluminum alloy. Moving on, XJet’s Carmel 1400 AM system was installed at KU Leuven University. Finally, Additive Minds investigated EOS 3D printing without the use of supports.
SCANLAB Integrating Process Data into 3D Printing
Laser scanning solutions manufacturer SCANLAB GmbH is at formnext 2019 this week, and will be introducing a scan system control extension that uses a smart data-acquisition interface that reads external sensors. It’s a functioning model of an intelligent interface, and can integrate sensor data into scan system control – giving AM users the ability to inquire about, and evaluate, centralized process data.
Two tradeshow demonstrators were created that show how diverse the integrable sensor range is. The first incorporates a surface-temperature pyrometer into the scan head control, and the sensor system’s data merges with laser beam position data. In the second, an OCT (optical coherence tomography) sensor from Precitec is integrated to measure the powder bed’s surface topography. Visit SCANLAB at formnext this week at Booth B41, Hall 12.0.
Desktop Metal Launches 4140 Chromoly Steel for Studio System
Massachusetts-based company Desktop Metal is expanding its material portfolio by launching 4140 chromoly steel for industrial applications for its office-friendly Studio System. 4140 is a versatile material, with high tensile strength, abrasion and impact resistance, and toughness. DM Studio Systems users can now use this material to 3D print parts like connecting rods, couplings, pinions, press brake tools, and more for industries including automotive, agriculture, industry, and defense.
“As global demand for the Studio System grows, Desktop Metal is broadening its materials portfolio to include 4140 chromoly steel, enabling designers and engineers to print a broad variety of critical industrial applications, such as couplings, forks, pinions, pump shafts, sprockets, torsion bars, worm gears, connecting rods, and fasteners. Now, teams around the world will be able to leverage the Studio System to iterate quickly on 4140 prototypes and ultimately produce end-use, customer-ready parts faster and more cost-effectively,” said Desktop Metal’s CEO and Co-Founder Ric Fulop.
QuesTek’s 3D Printable Aluminum Alloy
Integrated Computational Materials Engineering (ICME) technologies leader QuesTek Innovations LLC and the German Aerospace Center (DLR) are working on a joint project to explore the potential of QuesTek’s new 3D printable high-temperature aluminum (Al) alloy. The material, able to perform at temperatures between 200-300°C in its as-built condition, is being developed by QuesTek under several US Navy-funded Small Business Innovation Research awards, and is believed to be the first powdered Al alloy to meet necessary requirements without any subsequent heat treatment. The DLR will be 3D printing demonstration components with the material, which can be used to fabricate more lightweight precision components like heat exchangers.
“The accelerated design and development of a printable aluminum alloy capable of meeting so many current needs is especially exciting, as it will enable concurrent design of material composition and component geometry,” stated Greg Olson, QuesTek Chief Science officer. “Based on our internal test results, we see broad application of this material in manufacturing components for aerospace, satellite, automotive and high-performance racing.
“We are particularly pleased to be collaborating with the DLR. Their unrivaled reputation, expertise and close relationship with industry needs will bring an important new scope to our efforts.”
XJet’s Carmel 1400 3D Printer Installed at KU Leuven University
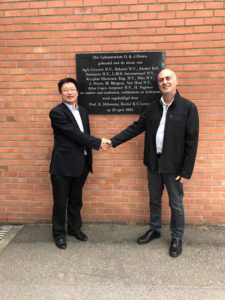
Professor Shoufeng Yang, KU Leuven, shakes hands with Avi Cohen, VP of Healthcare and Education at XJet.
For the first time, a 3D printing system has been installed at a European academic institution. XJet recently delivered its Carmel 1400 AM system to the KU Leuven University in Belgium, where it will be used to for university research and to help develop regional 3D printing medical opportunities. The 3D printer, and its proprietary NanoParticle Jetting (NPJ) technology, will be put to good use at the European research center, as academics will used it to explore medical applications and AM educational and research purposes. XJet’s zirconia material will also be used to 3D print ceramic medical models.
“Since the Carmel was installed, we are already reaping the benefits. The XJet system offers the high levels of precision and exceptional detailing required, levels which were previously impossible or extremely time-consuming in post-processing. The use of soluble support materials, with no harmful powders, makes it a much easier process and opens up opportunities to innovate that simply did not exist before,” said Professor Shoufeng Yang, who is heading the AM research at KU Leuven. “It’s an amazing and fantastic technology for R&D in universities and for the manufacturing industry, and it’s very exciting to be a part of. I believe that this is the best ceramic additive manufacturing method which can be easily upgraded into future multi-materials additive manufacturing, which is a grand challenge in the AM industry.”
XJet is also attending formnext this week – you can find the company at stand #C01 in Hall 12.1.
3D Printing Without Supports
Michael Wohlfart, DMLS Process Consultant for the EOS Additive Minds Process Consulting team, wrote an article on LinkedIn, titled “Building without support? Possibilities and limitations,” about the design aspect of printing without supports in metal powder bed fusion technology, which can reduce build time, material consumption, and cost. The three main reasons for supports are heat transfer, residual stress, and recoater forces, but there are workarounds for all three. In recoater forces, forces are acting on the part while spreading powder, and the recoater will wipe away parts not connected to the baseplate. Prop supports, such as cones and stacking parts, can be used to negate the need for a baseplate connection. Wolfhart discussed a few examples that were 3D printed on an EOS M290 out of titanium.
“Let’s move on to a more advanced design and even incorporate stacking,” Wohlfart wrote. “Since Christmas season is coming up, how about a Christmas tree designed with Siemens NX and pimped with nTopology? By turning it upside-down, the tree is self-supporting and the tree trunk can act as a shell for the next tree. You can see a small overlap of 0.1 mm in x-y-direction between the lattice and the solid parts in order to assure a good connection.”
To learn more, check out Wohlfart’s LinkedIn post.
Discuss these stories and other 3D printing topics at 3DPrintBoard.com or share your thoughts in the Facebook comments below.
The post 3D Printing News Briefs: November 20, 2019 appeared first on 3DPrint.com | The Voice of 3D Printing / Additive Manufacturing.