When it comes to medical applications, we’ve seen 3D printing used in the past for healing and repairing wounds, whether through the use of 3D printed bandages, 3D printed blood platelets, or bio-based materials, like nanocellulose. Researchers Dr. Mohamed M. Kanjou, Hassan Abdulhakim, Gabriel Molina de Olyveira, and Pierre Basmaji published a paper, titled “3-D Print Celulose Nanoskin: Future Diabetic Wound Healing,” about using bacterial cellulose for the purposes of wound healing.
“Most 3D printers use heat to melt the plastic or metal to be printed, and biobased materials are degraded,” the team wrote. “But cellulose nanofibrils have a solution to this problem: the printing paste is wet and dries out to a solid material. In this work, it was showed recent wound healing in Vinous Ulcer with kidney and other health complications using bacterial cellulose 3D print membranes.”
Cellulose nanofibrils, also known as nanocellulose, are made from wood or bacteria, and are the smallest fibers into which cellulose can be decomposed. They can contain up to 50% water, and this viscosity makes it ideal for a 3D printing paste, which can produce strong, biodegradable materials once they’ve dried out. By manipulating the cross-links between the fibrils, the properties can be modified, which allows for the fabrication of strong, porous, and flexible structures.
“Nanocellulose increases the opportunities for creating new materials in wound healing therapy. But this development still requires moisture tests to develops 3D printing with cellulose nanofibrils for medical and biotechnology applications,” the researchers explained.
“Several articles were published by our group since 2015 using Nanoskin membranes for wound healing treatment with successful results in diabetic ulcers, car and other accidents, amputation required ulcers [4] [5] [6]. In this work, it was showed recent wound healing in Vinous Ulcer with kidney and other health complications using bacterial cellulose 3D print.”

Wound healing treated with 3D bacterial cellulose-biological wound dressing (a); developed membrane (b) and Nanoskin developed equipament (c).
This time, the team explored a novel biomaterial and prepared a variety of different bacterial cellulose nanocomposites, such as BC/chondroitin sulfate and hyaluronic acid cross linked with sodium alginate and calcium chloride. They also synthesized bacterial cellulose and bacterial cellulose/chondroitin sulfate/hyaluronic acid.
“The acetic fermentation process was achieved by using glucose as a carbohydrate source,” the researchers explained. “Results of this process were vinegar and a nanobiocellulose biomass. The modifying process was based on the addition of hyaluronic acid and chondroitin sulfate (1% w/w) to the culture medium before bacteria inoculation. Bacterial cellulose (BC) was produced by Gram-negative bacteria Gluconacetobacter xylinus, which could be obtained from the culture medium in the pure 3-D structure, consisting of an ultra fine network of cellulose nanofibers.”
Dr. Kanjou and Abdulhakim supervised the completion of an in vivo analysis – the model was a 60-year-old patient diagnosed with a diabetic foot wound.
Here’s your warning – more icky wound pictures are coming.
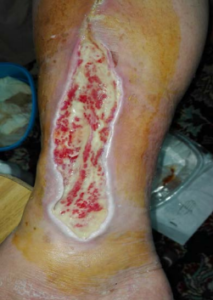
Wound healing evolution in 1 month and 3D bacterial cellulose impact use with biological wound dressing.
When the patient, also suffering from kidney failure, arrived at Sheikh Khalifa Hospital, the wound was infected and had accumulated a lot of slough tissue. A classic silver dressing did not show any progress, so the researchers began treating the patient’s wound with 3D printed bacterial cellulose membranes.
For one month, the 3D printed bacterial cellulose material was used on alternating days, to some excellent results – the edge and bottom of the wound were starting to heal, and the wound area was reduced.
Additionally, the slough tissue was easy to remove, and healthy red granulation tissue was starting to grow, which you can see in the below image.

Wound healing evolution in 2 months, 3D print Bacterial cellulose impact use in biological wound dressing.
“Then, after more 1 month, almost all slough tissue is removed by treating with 3-D print Bacterial cellulose only; granulation and building up of healthy tissue is coming up with approximation of skin and the wound is closing,” the researchers wrote.
“Finally, after 4 months of treatment, there is complete healing with minimizing the scar in wound area and able to decrease with time.”
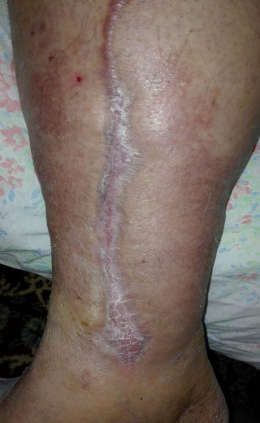
Figure 4. Complete wound healing evolution in 4 months and impact use of biological wound dressing 3D print of bacterial cellulose.
The researchers were able to successfully modify bacterial cellulose by “changing the fermentation medium with hyaluronic acid, chondroitin sulfate, besides of crosslinked with alginate sodium and calcium chloride.” In so doing, they were able to fabricate promising 3D printed scaffolds out of the bio-based material. In addition, the team developed new equipment for carrying out its work.
“In conclusion, 3-D print bacterial cellulose membranes apply to diabetic ulcers, with significant lesions and wound healing requirement; furthermore, natural membranes applications are for all population with different age.”
Discuss this research and other 3D printing topics at 3DPrintBoard.com or share your thoughts in the Facebook comments below.
The post Researchers Create 3D Printed Bacterial Cellulose Material for Wound Healing appeared first on 3DPrint.com | The Voice of 3D Printing / Additive Manufacturing.