Corals are dying globally. In the face of climate change and global warming, we can expect some severe consequences, which in turn directly affects marine life. In what is panning out to be a mass extinction event, coral reefs have been dangerously threatened by toxic substances and excess carbon dioxide for years, causing the certain death of may of these diverse marine invertebrates. Once the coral is dead, the reefs will also die and erode, destroying important marine life, that would otherwise feed and spawn on it.
Considering that scientists have predicted that nearly all coral reefs will disappear in 20 years, it is crucial that we protect corals and learn from them. For the expanding field of biotechnology, untapped resources like corals hold great potential, as bioactive compounds for cancer research or simply as an inspiration for the production of bioenergy and bioproducts.
In an interview with 3DPrint.com, interdisciplinary marine biologist Daniel Wangpraseurt, from the University of California San Diego (UCSD)’s Department of NanoEngineering, explained how bioprinting technology was a pivotal point in his work to develop bionic 3D printed corals as a new tool for coral-inspired biomaterials that can be used in algal biotechnology, coral reef conservation and in coral-algal symbiosis research.
“For many years I have been studying how corals optimize light management and discovered that there are lots of interesting evolutionary tricks, such as different growth forms and material properties, so I became interested in copying these strategies and developing artificial materials that could host living microalgae, just like corals do in nature,” revealed Wangpraseurt.
As one of the most productive ecosystems globally, coral reefs use photosynthesis to convert carbon dioxide into energy that they in turn use for food. Even though light provides the energy that fuels reef productivity, key nutrients such as nitrogen and phosphorus are also required, but are found in very low quantities in warm tropical oceans where coral reefs are generally found, making scientists wonder how these marine animals have managed to create a competitive habitat with such limited resources.
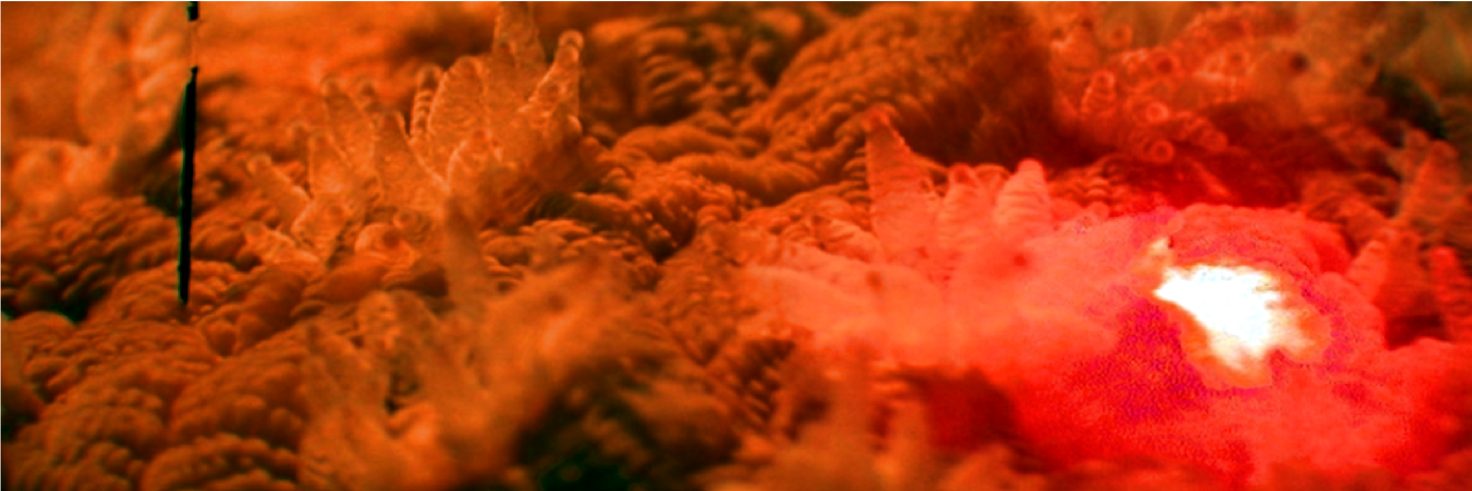
A laser beam is intensely scattered by elastic coral tissue and aragonite skeleton. (Credit: Daniel Wangpraseurt)
Wangpraseurt described that, while different corals have developed a plethora of geometries to achieve such capabilities, they are all characterized by an animal tissue-hosting microalgae, built upon a calcium carbonate skeleton that serves as mechanical support and as a scattering medium to optimize light delivery toward otherwise shaded algal-containing tissues.
“Taking what we learned about corals and biomaterials, we began working on a project to develop a synthetic, symbiotic system using a 3D bioprinting approach. We know corals have both animal cells and algal cells, and, so far, we have mimicked the animal part of the corals, that is, the physical and chemical microhabitat that partially controls the activity of the algal cells.”
At UCSD, Wangpraseurt expects to continue recreating coral-inspired photosynthetic biomaterial structures using a new bioprinting technique and a customized 3D bioprinter capable of mimicking functional and structural traits of the coral-algal symbiosis. Along with fellow researchers from UCSD, the University of Cambridge, the University of Copenhagen and the University of Technology Sydney, and thanks to a grant from the European Union’s Horizon 2020 research and innovation program, and the National Institutes of Health (NIH), the team reported the results of their work on bioinspired materials that was published in the journal Nature Communications earlier this year.
“We want to go further and not just develop similar physical microhabitat but also modulate cellular interactions, by mimicking biochemical pathways of symbiosis. We hope that this allows us to not only optimize photosynthesis and cell growth, but also to gain a deeper understanding of how the symbiosis works in nature. By doing so, we can improve our understanding of stress phenomena such as coral bleaching, which is largely responsible for global coral death.”

Living colonies of Symbiodinium are visible within the 3D bioprinted tissues (Credit: Daniel Wangpraseurt)
So, how did bioprinters become the go-to technology for this project? Wangpraseurt explains that, while working as a researcher at the University of Cambridge’s Department of Chemistry Bio-Inspired Photonics lab, he noticed that scientists were using cellulose as a biomaterial with interesting optical responses. He was wondering how he could use cellulose to develop a material with very defined architectural complexity.
“In the beginning, the main aim was to develop a coral-inspired biomaterial, that has a similar optical response as natural coral, and then to grow algae on it or within it. Thereby, we started off with simple techniques, using conventional 3D printers; however, it wasn’t very easy to recreate the spatial resolution we needed for corals.”
Inspired by 3D bioprinting research in the medical sciences, Wangpraseurt reached out to scientists at the UCSD NanoEngineering lab that were developing artificial liver models, and who later became collaborators in the project.
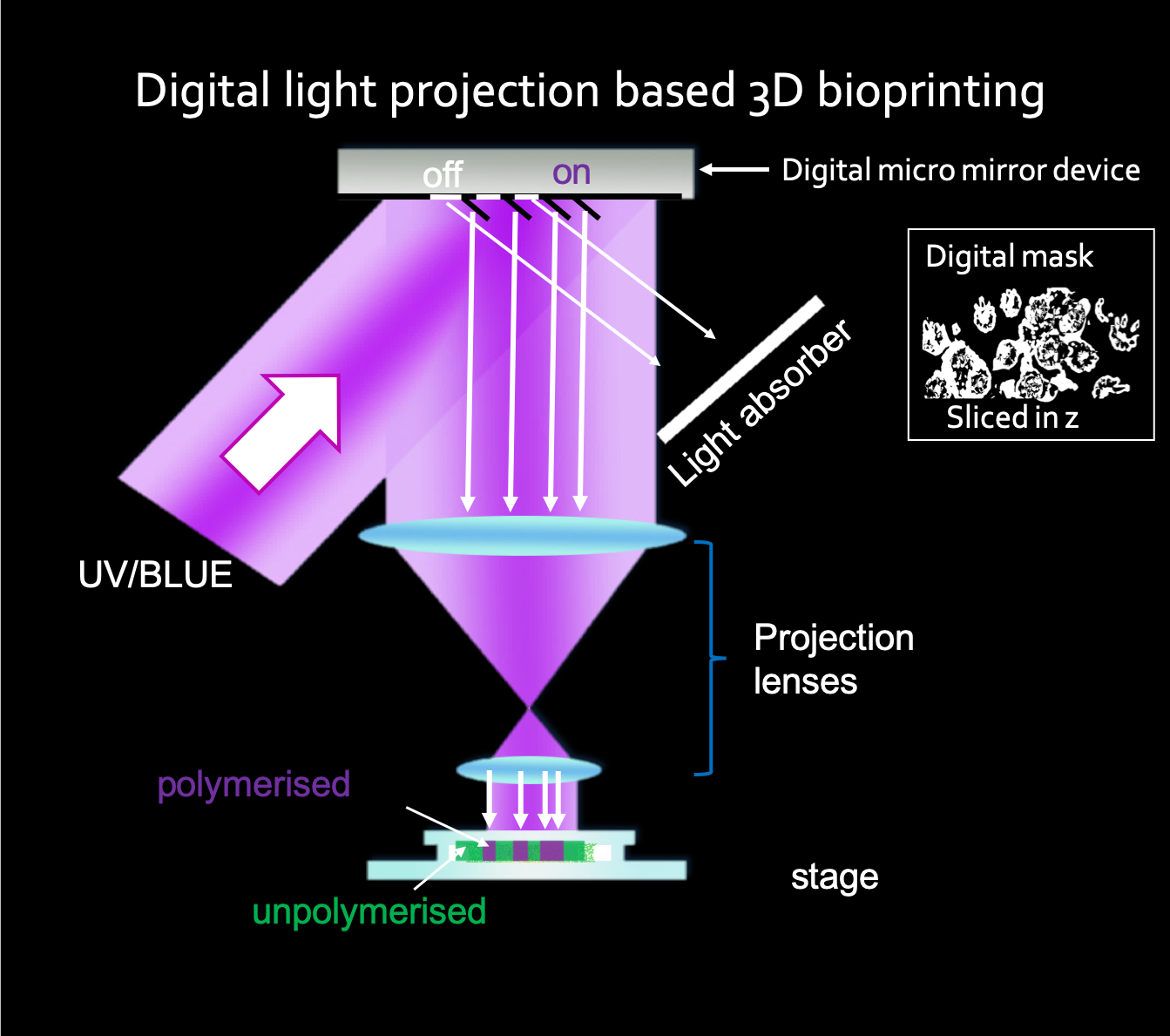
A laser beam is intensely scattered by elastic coral tissue and aragonite skeleton (Credit: Daniel Wangpraseurt)
The team went on to develop a 3D printing platform that mimics morphological features of living coral tissue and the underlying skeleton with micron resolution, including their optical and mechanical properties. It uses a two-step continuous light projection-based approach for multilayer 3D bioprinting and the artificial coral tissue constructs are fabricated with a novel bioink solution, in which the symbiotic microalgae are mixed with a photopolymerizable gelatin-methacrylate (GelMA) hydrogel and cellulose-derived nanocrystals (CNC). Similarly, the artificial skeleton is 3D printed with a polyethylene glycol diacrylate-based polymer (PEGDA).

Close up of coral polyps and living photosynthetic biomaterials. Living colonies of Symbiodinium are visible within the 3D bioprinted tissues (Credit: Daniel Wangpraseurt)
Based in San Diego, Wangpraseurt has spent months trying to recreate the intricate structure of the corals with a distinguished symbiotic system that is known to grow as it creates one of the largest ecosystems on the planet.
“We used a 3D bioprinter that had been developed for medical purposes, which we modulated and further developed a specific bioink for corals. A lot of the work was related to the optimization of the material properties to ensure cell viability. Having the right bioink for our algal strains was crucial as if we were to use mixtures commonly used for human cell cultures, the cells will not grow very well and can die rapidly.”
The implications of the newly developed 3D printed bionic corals capable of growing microalgae are many. Wangpraseurt said he plans to continue working on bionic corals and potentially scale up the process for his startup, called mantaz, as well as for commercial properties; or to develop coral-inspired materials at a larger scale to have a more immediate impact on efforts related to coral reef restoration, and also for biotechnology.
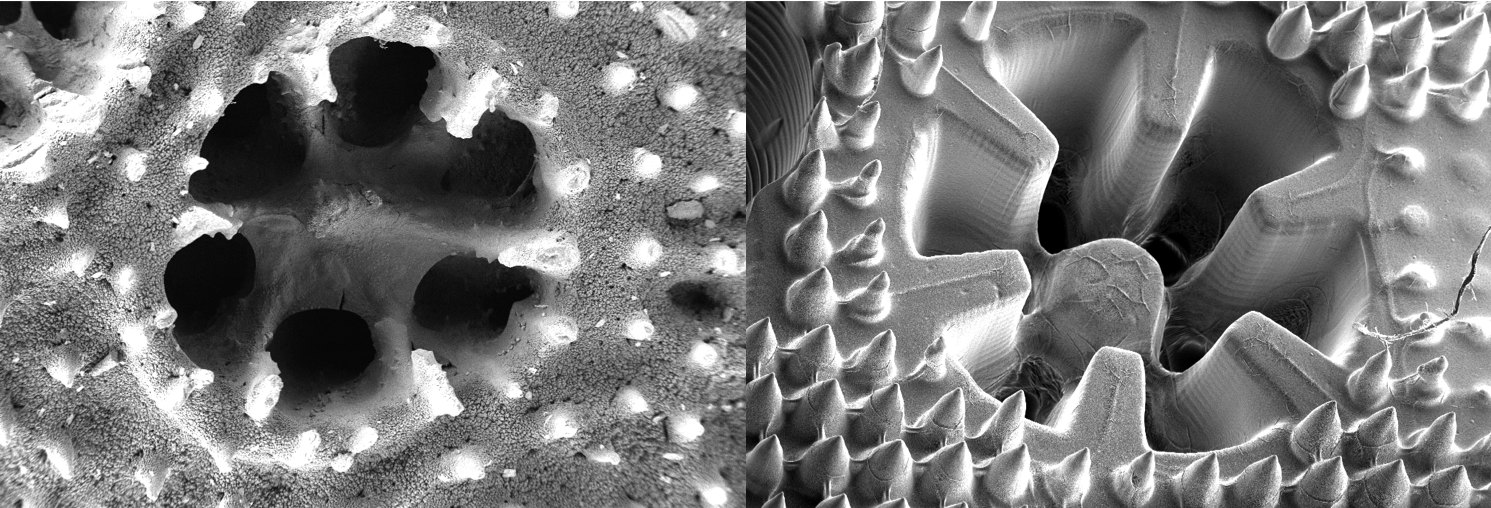
SEM images of the skeleton structure of the coral Stylophora pistillata and the coral-inspired, 3D-printed material (Credit: Daniel Wangpraseurt)
Wangpraseurt is looking to scale the bioprinting system to have a more immediate impact on algae biotechnology, bioenergy, and bioproducts. He claims that he and his colleagues can “customize the environment of the algae and fine-tune the production of a certain bioproduct to potentially tap into the algae bioproduct market and scale the system for bioenergy production.”
“Another interest of mine is to further develop a 3D bioprinted synthetic coral-algal symbiosis system, which can provide important insight into the mechanisms that lead to coral death, but can also result in the development of future technology for coral reef restoration.”
The researcher talks about coral reefs with a reverent passion that today goes beyond his lab work. When he is not moving the research along at USCD, Wangpraseurt is working with his social enterprise in Panama, as he and his team try to restore coral reef ecosystems to help coastal communities in the tropics, including local fishermen, by harvesting algae biomass that can be sold for different purposes, such as natural fertilizer, which contributes to an organic and sustainable chain of production. Furthermore, the coral-inspired aspects of Wangpraseurt’s research and startup company are really coalescing to enable him and his team to understand how corals work and, in turn, how we can learn from them for the benefit of our planet.
The post Marine Biologist Modifies Bioprinting for the Creation of Bionic Coral appeared first on 3DPrint.com | The Voice of 3D Printing / Additive Manufacturing.