Delft researchers formulate novel calcined clay-based cement for 3D printing
Oregon researchers develop lego-inspired 3D printed healing ‘bone bricks’
University of California San Diego researchers use 3D printing to create “insect-like” soft robots
University of Illinois researchers create ‘walking’ biobot by combining 3D printed hydrogel skeleton with rat spinal cord
Prize winning space-time research expands design for additive manufacturing
Delft University of Technology & Maaike Roozenburg 3D Print Chinese Porcelain
China is famous for its blue and white porcelain, delicately and artfully produced and painted. Crafted mainly in the southern Chinese city of Jingdezhen and purchased by travelers visiting the East Asian markets, this porcelain also served as a popular export—from the 1600s on; in fact, up to 35 million pieces may have been exported by the Dutch East India Company. Now, designer Maaike Roozenburg is working with Delft University of Technology, not only to preserve an important heritage but also to bring forth beautiful 3D samples of originals for everyone to enjoy. A few years ago 3D printing maven Olivier van Herpt developed 3D printing for porcelain and the world’s first porcelain 3D printed pieces can be seen in The Gemeentemuseum in The Hague. Now Maaike and the University of Delft have come up with a way to scan and recreate ancient objects.
“The whole history of porcelain is fascinating,” says Roozenburg. “It’s this magical white material and something they invented in China. There was an obsession with it. Everyone wanted it, and in China, they started to really produce porcelain especially for western markets. All the royals in Europe loved it.”
Artists in the Netherlands were particularly fond of the Chinese porcelain and its classic design, and they created Delftware, an imitation which became its own brand.
“In the 17th century, ceramic Delftware vases decorated the gardens of royal Het Loo Palace in Apeldoorn,” Roozenburg says. “But they are all gone. Archeologists found some bits and pieces, and scientific proof that they were there, together with drawings and paintings.”
“Thank God, at the time, royals gave these vases as presents to other royals!” says Roozenburg, commenting on the fact that only two were found—one in Erddig Hall in the UK, and the other in the museum of Schloss Favorite in Germany.
Unfortunately, administrators would not allow any vases to be taken out of the respective castles, so Roozenburg and his team traveled to them, bearing Artec 3D scanners in their backpacks and overcoming a variety of challenges, including scanning problems due to the reflective nature of the glass (overcome with the performance of the Artec Eva scanner).
The team also made practice vases first, using Styrofoam models to practice on:
“You don’t want any surprises when you’re in a castle in Britain and realize you have to improvise!” said Roozenburg.
“I think what’s amazing about scanning is that, by rendering physical objects that are really fragile, valuable, and have to stay within a museum into digital data, you can experiment with them,” says Roozenburg. “It gives new opportunities to work with historical objects that you otherwise would not be able to touch.”
Because handling of the objects is such a major issue due to worries over disintegration or items being broken, they created a round platform with a small motor—allowing the vase to be rotated and scanned via the Artec Eva, mounted on a tripod. Next, they used the Artec Space Spider for detailed ornamental parts like lions heads and shells. Once scanned, files were processed in MATLB and then 3D printed.
“The best thing is, in the end you can’t see that there was any new tech used. The finished work of 45 vases look like the originals, like they are brand new coming from the oven in the 17th century,” says Roozenburg.
With 3D scanning, users around the world are able to enjoy the benefit of making parts that need to be replaced, or that in some cases have become obsolete; here, everyone involved in the project is able to work with pieces that are hundreds of years old—and able to be handled without worry once they are transformed into 3D form.
“It’s culture,” says Technical University scanning expert Naagen. “We should make it accessible to people all over the world.”
Scanning equipment from Artec3D has been behind some of the most exciting projects involving artifacts over the past few years, involving archiving work with museums, preserving the first World War 1 monument, and much more. What do you think of this news? Let us know your thoughts! Join the discussion of this and other 3D printing topics at 3DPrintBoard.com.
[Source / Images: Artec 3D]
The post Delft University of Technology & Maaike Roozenburg 3D Print Chinese Porcelain appeared first on 3DPrint.com | The Voice of 3D Printing / Additive Manufacturing.
3D Printing News Sliced: Makerbot, 3D Systems, GE Additive, EOS, ColorFabb, TU Delft, SUTD, AMT
TU Delft engineers develop self-aware 3D printed soft robots
Collaborative Research Team Develops Density-Graded Structure for Extrusion 3D Printing of Functionally Graded Materials

Microscopic photos of top and side views of printing results with a 0.38 mm wide extrusion path: (a) without versus (b) with overlapping by 0.36 mm respectively. Overlapping extrusion paths exhibit over-extrusion of material at the overlapping region, which results in unwanted blobs on the surface of the print.
Plenty of research has been completed in regards to FDM (extrusion) 3D printing, such as how to improve part quality and how to reliably fabricate functionally graded materials (FGM). The latter is what a collaborative team of researchers from Ultimaker, the Delft University of Technology (TU Delft), and the Chinese University of Hong Kong are focusing on in their new research project.
The team – made up of researchers Tim Kuipers, Jun Wu Charlie, and C.L. Wang – recently published a paper, titled “CrossFill: Foam Structures with Graded Density for Continuous Material Extrusion,” which will be presented at this year’s Symposium for Solid and Physical Modeling.
“In our latest paper we present a type of microstructure which can be printed using continuous extrusion so that we can generate infill structures which follow a user specified density field to be printed reliably by standard desktop FDM printers,” Kuipers, a Software Engineer and Researcher for Ultimaker, wrote in an email.
“This is the first algorithm in the world which is able to generate spatially graded microstructures while adhering to continuous extrusion in order to ensure printing reliability.”
Because 3D printing offers such flexible fabrication, many people want to design structures with spatially graded material properties. But, it’s hard to achieve good print quality when using FDM technology to 3D print FGM, since these sorts of infill structures feature complex geometry. In terms of making foam structures with graded density using FDM, the researchers knew they needed to develop a method to generate “infill structures according to a user-specific density distribution.”
The abstract reads, “In this paper, we propose a new type of density graded structure that is particularly designed for 3D printing systems based on filament extrusion. In order to ensure high-quality fabrication results, extrusion-based 3D printing requires not only that the structures are self-supporting, but also that extrusion toolpaths are continuous and free of self-overlap. The structure proposed in this paper, called CrossFill, complies with these requirements. In particular, CrossFill is a self-supporting foam structure, for which each layer is fabricated by a single, continuous and overlap-free path of material extrusion. Our method for generating CrossFill is based on a space-filling surface that employs spatially varying subdivision levels. Dithering of the subdivision levels is performed to accurately reproduce a prescribed density distribution.”
Their method – a novel type of FDM printable foam structure – offers a way to refine the structure to match a prescribed density distribution, and provides a novel self-supporting, space-filling surface to support spatially graded density, as well as an algorithm that can merge an infill structure’s toolpath with the model’s boundary for continuity. This space-filling infill surface is called CrossFill, as the toolpath resembles crosses.
“Each layer of CrossFill is a space-filling curve that can be continuously extruded along a single overlap-free toolpath,” the researchers wrote. “The space-filling surface consists of surface patches which are embedded in prism-shaped cells, which can be adaptively subdivided to match the user-specified density distribution. The adaptive subdivision level results in graded mechanical properties throughout the foam structure. Our method consists of a step to determine a lower bound for the subdivision levels at each location and a dithering step to refine the local average densities, so that we can generate CrossFill that closely matches the required density distribution. A simple and effective algorithm is developed to merge a space-filling curve of CrossFill of a layer into the closed polygonal areas sliced from the input model. Physical printing tests have been conducted to verify the performance of the CrossFill structures.”
The researchers say that the user prescribes density distribution, and can use CrossFill and its space-filling surfaces, with continuous cross sections, to “reliably reproduce the distribution using extrusion-based printing.” CrossFill surfaces are built by using subdivision rules on prism-shaped cells, each of which contains a surface patch that’s “sliced into a line segment on each layer to be a segment” of the toolpath, which will be made with a constant width; cell size determines the density.
“By adaptively applying the subdivision rules to the prism cells, we create a subdivision structure of cells with a density distribution that closely matches a user-specified input,” the team wrote. “Continuity of the space-filling surface across adjacent cells with different subdivision levels – both horizontally and vertically – is ensured by the subdivision rules and by post-processing of the surface patches in neighboring cells.”
The subdivision system distinguishes an H-prism, which is built by cutting a cube in half vertically along a diagonal of the horizontal faces, and a Q-prism, generated by spitting a cube into quarters along the faces’ diagonals. To learn more about this system and the team’s algorithms, check out the paper in its entirety.
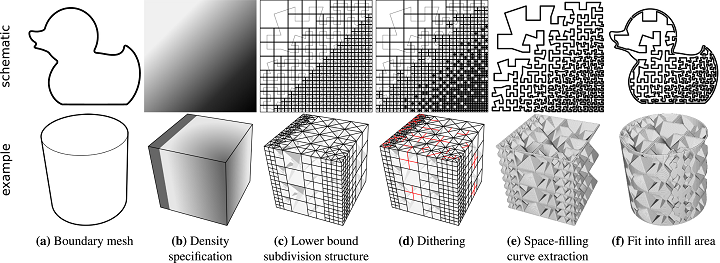
Schematic overview of our method. The top row shows a 2D analogue of our method for clear visualization. The prism-shaped cells in the bottom row are visualized as semi-opaque solids to keep the visualization uncluttered. Red lines in the bottom row highlight the local subdivisions performed in the dithering phase.
The researchers also explained the method’s toolpath generation in their paper, starting with how to slice the infill structure into a continuous 2D polygonal curve for each layer of the object, which is followed by fitting a layer’s curve “into the region of an input 3D model.”
Experiments measuring features like accuracy, computation time, and elastic behavior were completed on an Intel Core i7-7500U CPU @ 2.70 GHz, using test structures 3D printed out of white TPU 95A on Ultimaker 3 systems with the default Cura 4.0 profile of 0.1 mm layer thickness. The team also discussed various applications for CrossFill, such as imaging phantoms for the medical field or cushions and packaging.
“The study of experimental tests shows that CrossFill acts very much like a foam although future work needs to be conducted to further explore the mapping between density and other material properties,” the researchers concluded. “Another line of research is to further enhance the dithering technique, e.g. changing the weighing scheme of error diffusion.”

CrossFill applications. (a) Bicycle saddle with a density specification. A weight of 33 N is added on various locations to show the different response of different density infill. (b) Teddy bear with a density specification. (c) Shoe sole with densities based on a pressure map of a foot. (d) Stanford bunny painted with a density specification. (e) Medical phantom with an example density distribution for calibrating an MRI scanning procedure.
The team’s open source implementation is available here on GitHub. To learn more, check out their video below:
Discuss this story, and other 3D printing topics, at 3DPrintBoard.com or share your thoughts below.