Creality CR-6 SE Review // Should You Back It On KICKSTARTER?
FDM 3D Printing: Effects of Typical Parameters on Functional Parts
Ahmed Elkaseer, Stella Schneider, and Steffen G. Scholz delve further into the effects of parameters and settings on the quality of parts, releasing the details from their recent study in ‘Experiment-Based Process Modeling and Optimization for High-Quality and Resource-Efficient FFF 3D Printing.’
Reminding us that 3D printing was originally designed for rapid prototyping, centered around the work of engineers, the authors point out that users around the world are now also beginning to rely on such technology for ‘customized mass production of functional parts,’ due to a sufficient evolution of the processes; however, there is still much to be learned and improved. This is especially true as users continue to innovate within so many different applications, from automotive to aerospace to construction, and so much more.
This study focuses on FDM (FFF, Material Extrusion) 3D printing as the authors examine what is accurately depicted as ‘a large number of individually adjustable printing parameters,’ and why defects and problems so often occur. Noting that PLA is a popular material in 3D printing, enjoyed due to its more environmentally friendly nature and ease in use, the authors analyzed the following on a test structure:
- Infill percentage
- Layer thickness
- Printing speed
- Printing temperature
- Surface inclination angle
Simplify3D was used as slicing software, with test samples 3D printed on the Zmorph 2.0 SX.
The authors reported that for this study, printing parameters remained the same for all experiments.
Three samples were 3D printed, allowing the researchers to average the process responses, measure the importance of the process responses, evaluate accuracy, and calculate percentage errors.
“For the surface roughness, Ra, the stylus-type profilometer MarSurf GD 26 was used,” stated the authors. “A sample length of 4 mm was traversed by the stylus. For each run, the roughness at the concerning inclination angle was measured at three samples and the average was calculated.”
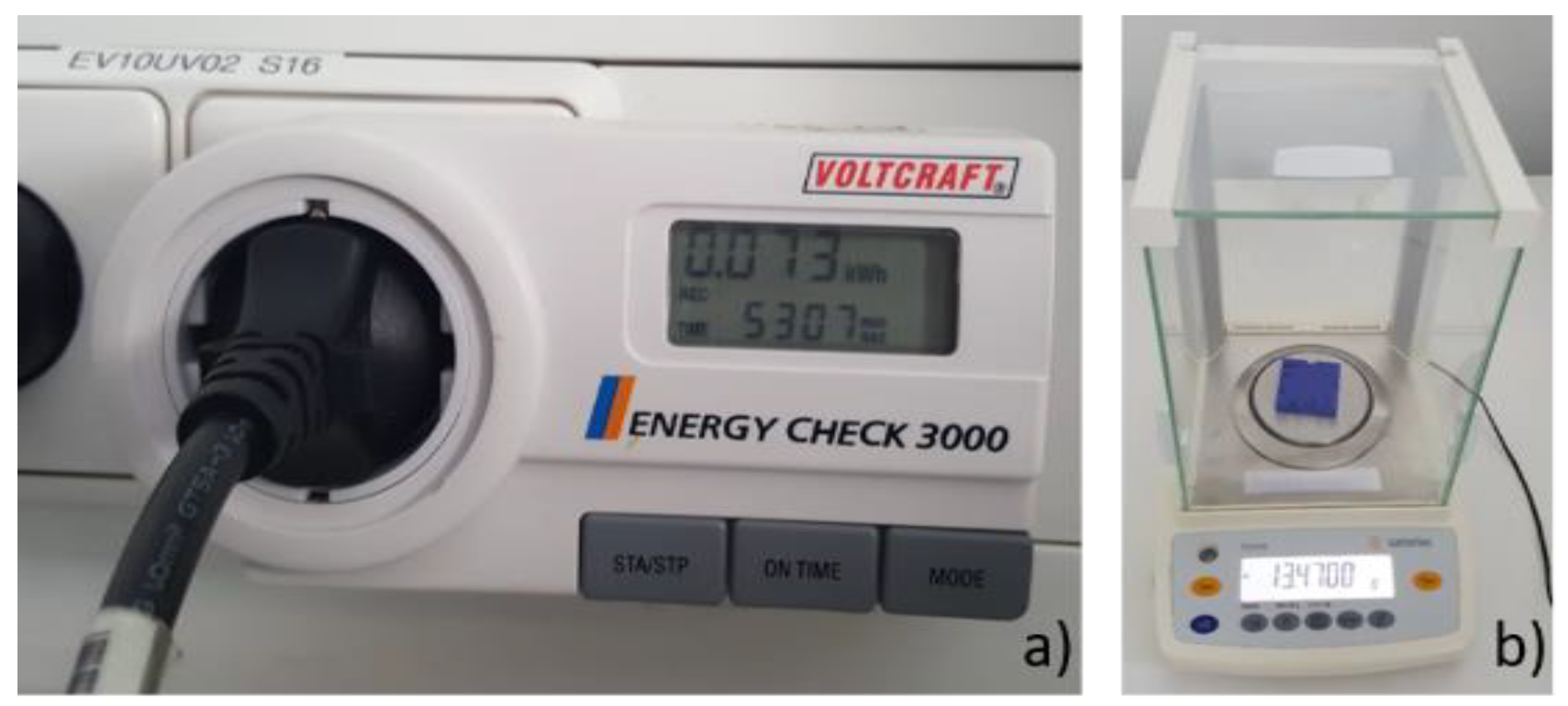
(a) Energy measuring device Voltcraft Energy Check 3000 and (b) analytical balance Sartorius Extend ED224S.
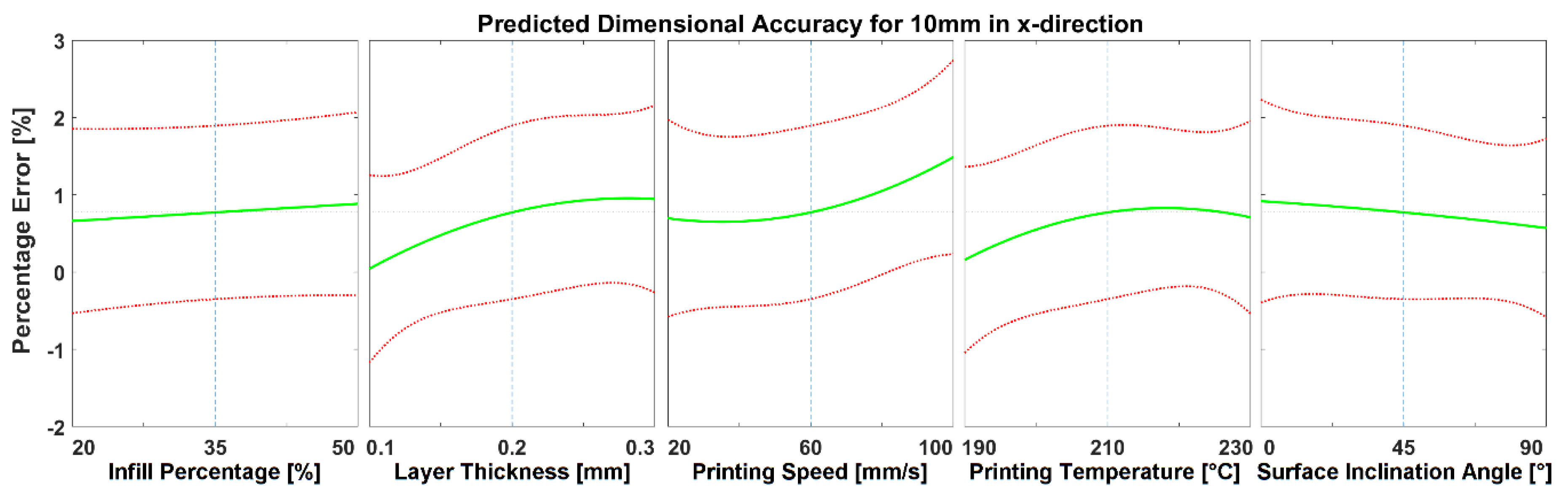
Predicted effects of changes in process parameters on the error% for dimensional accuracy of 10 mm length in the X direction.
The effects of the parameters used were analyzed in relation to:
- Surface roughness
- Dimensional accuracy
- Productivity
- Energy consumption

Predicted effects of changes in process parameters on the error% for dimensional accuracy of 10 mm length in the Z direction.
“Considering all the parameters, dimensional accuracy is mainly influenced by layer thickness and printing speed, whereas the surface roughness depends on surface inclination angle and on layer thickness. Energy consumption and productivity are primarily affected by printing speed and layer thickness. Although interactions between printing parameters can be beneficial for the required outcome, they can also hamper the process,” concluded the researchers.
“Generally, it is apparent that layer thickness and printing speed dominate the other parameters in their importance and usually define the outcome of the printing process. However, on the other hand, there will be a trade-off between layer thickness and printing speed to ensure part quality and resource usage, which must be resolved in order to obtain high-quality and resource-efficient built parts.”
What do you think of this news? Let us know your thoughts! Join the discussion of this and other 3D printing topics at 3DPrintBoard.com.
[Source / Images: ‘Experiment-Based Process Modeling and Optimization for High-Quality and Resource-Efficient FFF 3D Printing’]
The post FDM 3D Printing: Effects of Typical Parameters on Functional Parts appeared first on 3DPrint.com | The Voice of 3D Printing / Additive Manufacturing.
Researchers Evaluate Feasibility of Closing Multiple Atrial Septal Defects Guided by 3D Printed Model
We’ve often seen physicians use 3D printed heart models to help during surgeries, but a group of researchers from China published a paper on using them to help with an alternative to surgery for repairing secundum atrial septal defect (ASD), a rare congenital defect characterized by a hole in the wall between the atria. Their goal was to evaluate how feasible it was to use a single device to close several ASDs guided by the 3D printed heart model and transthoracic echocardiography (TTE).
Due to interference between devices and threat of repeat intervention, it’s difficult to use multiple devices simultaneously, or in staged device closure, in percutaneous transcatheter closure of an ASD. But using an over-sized device, can tear the atrial septum. So the best plan is to use single device closure for patients with multiple ASDs, as it preserves the anatomical structure.
“However, this strategy is technically challenging because of inability to determine the target defect for catheter passage and occluder selection, warranting careful interventional planning with comprehensive anatomical information for successful device closure,” the team wrote.
That’s where the 3D printed heart model comes in. The researchers used the single-device strategy, assisted by 3D printing, to perform multiple ASDs closure, and compared their results of “3D printing-based and transthoracic echocardiography (TTE)-guided percutaneous transcatheter closure with those of traditional fluoroscopy-guided closure.”

Simple working flowchart in patients with multiple ASDs, from image acquisition to 3D printed solid and hollow model.
62 patients diagnosed by TTE with two or more ASDs with a 5mm or more diameter, were enrolled in their non-randomized study for analysis. 30 had cardiac computed tomography angiography (CTA) ahead of surgery in order to get data to create their 3D printed heart models. The CTA images were reconstructed and saved in DICOM format, before being imported to Materialise Mimics software. Cardiac masks were generated for 3D models, and 3-matic software was used to hollow them. The STL files were 3D printed, in hollow fashion, at 1:1 scale on a ProJet MJP 2500 Plus 3D printer out of silicone.

3D printed model of a patient with multiple ASDs. (a) and (b) show the model from left and right atrial sides, respectively. The arrows depict the position of the ASDs. (c) and (d) illustrate the status after occluder deployment in the model.
The surgeons performed in vitro simulated occlusion with the 3D printed models as a pre-op evaluation. Then, while the other 32 patients underwent ASD closure with fluoroscopic guidance, this group had TTE-guided closure procedures.
“The apical four-chamber view and parasternal short-axis view were used for guidance, and the multipurpose catheter was passed through the targeted defect, which was determined using the 3D printing model and intraoperative TTE,” the researchers explained.
“Then, a single septal occluder was inserted for ASD closure under TTE guidance. An ASD occluder or PFO occluder was selected based on the in vitro simulated occlusion in a 3D printing model.”
After implantation, the device position was evaluated through subcostal, apical four-chamber, and parasternal short-axis views, and they also performed Color Doppler assessment to detect any issues, like coronary sinus return or residual shunting. Once they determined that the occluder had been implanted correctly, “it was released by rotating the cable counterclockwise under TTE guidance,” and a reassessment was then performed in echo views, below.
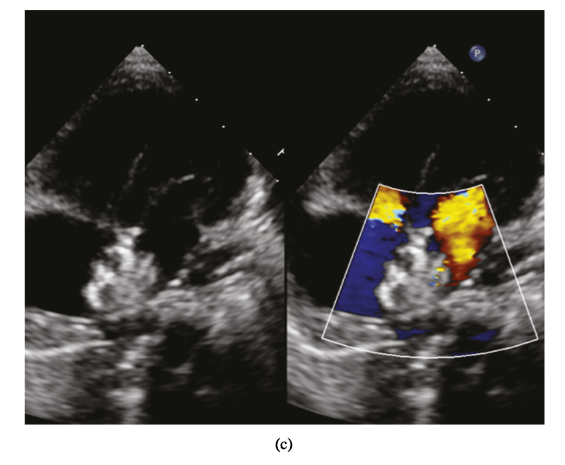
Percutaneous closure of multiple ASDs under TTE guidance. (a) Multiple ASDs image displayed in subcostal view. (b) The left disc was released (parasternal short-axis view). (c) The ASDs were closed (four-chamber view).
“In the conventional group, multiple ASDs occlusion was performed under fluoroscopic guidance using the single occlusion device,” they wrote. “Based on TTE measurements, the single device was selected, equal to or up to 4 mm larger than the main defect [10]. According to experience [10, 20, 21], the device was usually implanted into the largest defect. The occluder was replaced if echography found more than two residual shunts, the residual shunt was >5 mm in diameter, or the device compressed the mitral valve.”
Immediately post-op, and 6 months after the device closure, all 62 patients were evaluated via TTE and electrocardiogram, with the researchers noting the presence of any arrhythmia, residual shunt, or valve dysfunction. A Brand-Altman analysis was used to evaluate the agreement “between device size of 3D printed model and traditional estimation,” and the data was analyzed with SPSS software.

Bland–Altman plot analysis. Bland–Altman plot of empirical estimation versus 3D printed model estimation of occluder size.
They found that 26 patients in the 3D printing/TTE group, and 27 patients in the conventional group, achieved successful transcatheter closure with a single device. The prevalence of residual shunts was lower in the first group immediately and 6 months post-op, and there were no complications in either group during the procedure or the two follow-ups.
“Gender, age [18.8 ± 15.9 (3–51) years in the 3D printing and TTE group; 14.0 ± 11.6 (3–50) years in the conventional group], mean maximum distance between defects, prevalence of 3 atrial defects and large defect distance (defined as distance ≥7 mm), and occluder size used were similarly distributed between groups,” the team wrote. “However, the 3D printing and TTE group had lower frequency of occluder replacement (3.8% vs 59.3%, ), prevalence of mild residual shunts (defined as <5 mm) immediately (19.2% vs 44.4%, ) and at 6 months (7.7% vs 29.6%, ) after the procedure, and cost (32960.8 ± 2018.7 CNY vs 41019.9 ± 13758.2 CNY, ).”
They did note that the occluder on the 3D printed model was “consistently larger than in the empirical estimation but similar to final clinical selection,” which indicates a higher level of accuracy. Even in patients with a large defect distance, the results of the study suggest that “interventional therapy with a single occluder for multiple ASDs is feasible,” especially as technical difficulties and complex anatomy make successful single device closure tricky to achieve. It’s important to remember that the accuracy of the 3D printed anatomic model is paramount in attaining single device closure in patients with multiple ASDs.
“Occluders’ sizes preestimated by the 3D printed model were similar to the size actually used for patients and larger than the size from conventional empirical estimation. These results indicate that preevaluation using the 3D printed model can avoid unnecessary interventions, the possibility of enlarging ASD by changing occluders and the financial waste of replacing occluders,” they explained.
The researchers ultimately determined that it’s feasible to use a 3D printed model to help achieve successful device closure for patients with multiple ASDs with a defect distance of ≥7 mm. The model can also help screen patients who may not be well-suited for the closure route, and should instead seek direct surgical repair.
“The combination of the 3D printing technology and ultrasound-guided interventional procedure provides a new approach for individualized therapeutic strategy of structural heart disease and in particular a reliable therapeutic method for multiple ASDs, especially for challenging cases with large defect distance,” they concluded.
Discuss this research and other 3D printing topics at 3DPrintBoard.com or share your thoughts below.
The post Researchers Evaluate Feasibility of Closing Multiple Atrial Septal Defects Guided by 3D Printed Model appeared first on 3DPrint.com | The Voice of 3D Printing / Additive Manufacturing.
Aalto University Develops a Novel Bioink for Cardiac Tissue Applications
Finland is one of Europe’s most forested nations. Over 70 percent of the country’s boreal forest is covered with spruce, pine, downy birch, and silver birch. But beyond the splendor of the Finnish woodlands, all these trees have one thing in common, and that is nanocellulose. A light solid substance obtained from plant matter which comprises cellulose nanofibrils (CNF) and is considered a pseudo-plastic that possesses the property of specific kinds of gels that are generally thick in normal conditions. Overall, it is a very environmentally friendly and non-toxic substance that is compatible with the human body and has the potential to be used for a range of medical applications.
In 2018, the Department of Bioproducts and Biosystems at Aalto University, located just outside Helsinki, began searching for new ideas to revitalize one of the country’s traditional economic engines, forests (which are handled sustainably thanks to renewable forest resources). At the time, they noticed that one of the possible applications could be working with nanocellulose. Forward two years and the researchers have come up with a new bioink formulation praising nanocellulose at its basis.
Thanks to the structural similarity to extracellular matrices and excellent biocompatibility of supporting crucial cellular activities, nanocellulose-based bioprinting has clearly emerged for its potential in tissue engineering and regenerative medicine. The qualities of the generally thick and fluid light substance make it an excellent match to develop bioinks that are both suitable and scalable in their production, but also have consistent properties. However, there have been major challenges in processing nanocellulose.
As described by Aalto University researchers in a recently published paper in the science journal ACS Publication, the unresolved challenges of bioink formulations based on nanocelluloses are what stops the substance from becoming one of the preferred components for 3D bioprinting structures. This is why Finnish researchers focused on developing a single-component bioink that could be used to create scaffolds with potential applications in cardiac biomedical devices, while fundamentally dealing with some of the limitations of using nanocellulose-based bioinks.
A co-author of the paper and a doctoral candidate at Aalto’s Department of Bioproducts and Biosystems, Rubina Ajdary, told 3DPrint.com that “other than natural abundance and as a renewable resource, nanocellulose has demonstrated to have an outstanding performance in tissue engineering.” She also suggested that “recent efforts usually consider the use of nanocellulose in combination with other biopolymers, for example, in multicomponent ink formulations or to encapsulate nanoparticles. But we were interested in investigating the potential of monocomponent nanocellulose 3D printed scaffolds that did not require crosslinking to develop the strength or solidity.”
“Most modifications make the hydrogels susceptible to dimensional instability after 3D printing, for instance, upon drying or wetting. This is exacerbated if the inks are highly diluted, which is typical of nanocellulose suspensions, forming gels at low concentrations,” went on Ajdary. “This instability is one of the main reasons why nanocellulose is mainly combined with other compounds. Instead, in this research, we propose heterogeneous acetylation of wood fibers to ease their deconstruction into acetylated nanocellulose for direct ink writing. A higher surface charge of acetylated nanocellulose, compared to native nanocellulose, reduces aggregation and favors the retention of the structure after extrusion even in significantly less concentration.”
“3D structures of acetylated nanocellulose are highly stable after extrusion in far less concentrations. The lower concentration in wet condition facilitates the scaffold with higher porosity after dehydration which can improve the cell penetration in the structure and assist in nutrient transport to the cells as well as in the transport of metabolic waste,” specified Ajdary.
The post Aalto University Develops a Novel Bioink for Cardiac Tissue Applications appeared first on 3DPrint.com | The Voice of 3D Printing / Additive Manufacturing.
Texas A&M Researchers: Eliminating the Weak Spots in 3D Printing
Researchers at Texas A&M, working with scientists from Essentium, Inc., continue to refine 3D printing processes. Pinpointing one of the most vulnerable areas, the individual printed layers, the researchers and scientists have developed new technology for improving part reliability with plasma science and carbon nanotube technology.
Upon the inception of 3D printing in the 80s with the SLA-1, such technology was primarily used for prototyping; however, in the past few decades, organizations like NASA , the military, and GE have begun to focus on fabrication of not only prototypes but also critical parts. The medical realm has been heavily impacted too. The area of prosthetics, for instance, is being completely transformed as groups like e-NABLE make it possible for individuals in need to receive affordable, completely customized limb replacements—around the globe.
Users on every level are taking advantage of the benefits of 3D printing, from affordability and speed in production, to the ability to cut out the middleman and innovate at will—whether on the industrial level, or in the office or at home. For many, thermoplastics such as ABS and PLA are still the most popular choices for fabrication. Layer bonding and issues with mechanical properties are ongoing challenges for most users, and while a defective prototype can be easily dealt with, an industrial part that fails could be disastrous.
“Finding a way to remedy the inadequate bonding between printed layers has been an ongoing quest in the 3D printing field,” said Dr. Micah Green, associate professor in the Artie McFerrin Department of Chemical Engineering. “We have now developed a sophisticated technology that can bolster welding between these layers all while printing the 3D part.”
The research team released the details of their study in the recently published, ‘Dielectric Barrier Discharge Applicator for Heating Carbon Nanotube-Loaded Interfaces and Enhancing 3D Printing Bond Strength.’ Their paper highlights the need for increased tensile strength and ‘production-ready solutions.’
Using a dielectric barrier discharge (DBD) plasma electrode that can be mounted on the 3D printer for welding of parts, the researchers were able to create parts strong enough to compare with traditionally created injection-molded parts.
In coating layers with carbon nanomaterials, the researchers could apply heat, thus causing the 3D printed layers to bond cohesively.
“If you put something in an oven, it’s going to heat everything, so a 3D-printed part can warp and melt, losing its shape,” said Green. “What we really needed was some way to heat only the interfaces between printed layers and not the whole part.”
The researchers began working with Dr. David Staack, associate professor in the J. Mike Walker ‘66 Department of Mechanical Engineering, creating conductive materials to charge the surfaces of the 3D prints, continuing to heat the materials, and ‘weld’ the materials in place
“The holy grail of 3D printing has been to get the strength of the 3D-printed part to match that of a molded part,” said Green.
“In this study, we have successfully used localized heating to strengthen 3Dprinted parts so that their mechanical properties now rival those of molded parts. With our technology, users can now print a custom part, like an individually tailored prosthetic, and this heat-treated part will be much stronger than before.”
The primary author for the research is Dr. C. Brandon Sweeney, a former Texas A&M materials science and engineering student in Green’s laboratory. He is the head of research and development and cofounder at Essentium.
Other contributors of this research include Dr. Blake R. Teipel ‘16 and Dr. Bryan S. Zahner ‘14 from Essentium; Dr. Martin J. Pospisil ’19, Dr. Smit A. Shah ’19, and Muhammad Anas from the Texas A&M chemical engineering department; and Matthew L. Burnette from the Texas A&M mechanical engineering department.
What do you think of this news? Let us know your thoughts! Join the discussion of this and other 3D printing topics at 3DPrintBoard.com.
[Source / Images: Texas A&M University]
The post Texas A&M Researchers: Eliminating the Weak Spots in 3D Printing appeared first on 3DPrint.com | The Voice of 3D Printing / Additive Manufacturing.
Additive Industries CEO Daan Kersten Steps Down as Firm Receives $14M Investment
One of a newer generation of metal laser powder bed fusion (PBF) manufacturers, Additive Industries is continuing to grow rapidly. The latest news is a $14 million investment from its existing shareholder, Highlands Beheer. With the funds, the company aims to expand its product portfolio, speed up its technological development strategy and shore up its working capital. This last use for the investment is meant to ensure financial resilience for the company amid the COVID-19 pandemic.
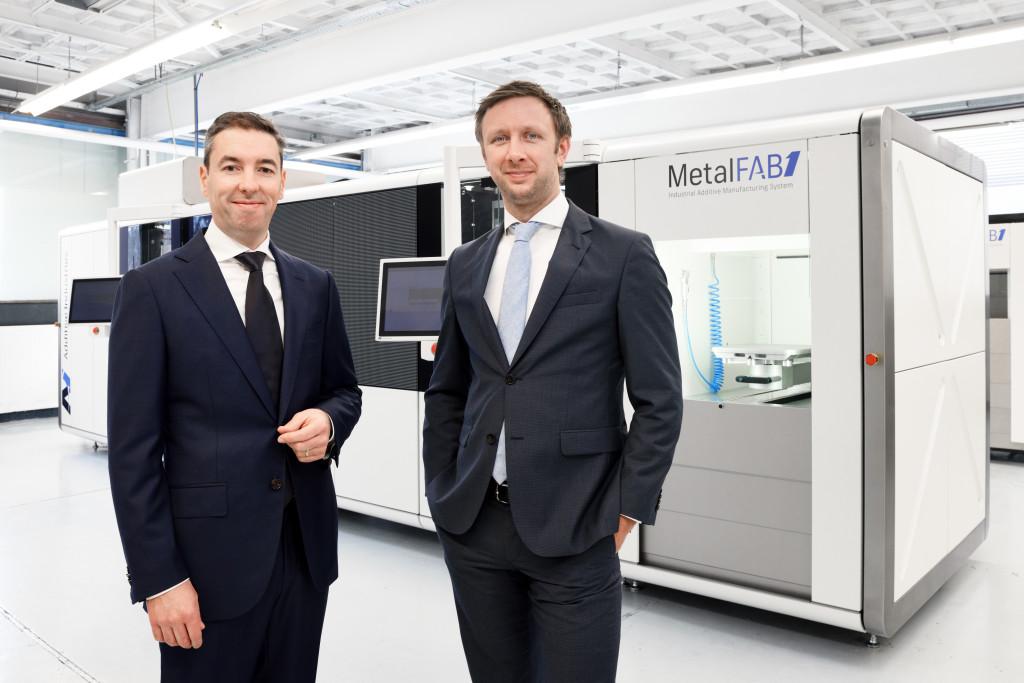
Outgoing Additive Industries CEO Daan Kersten (L) with Jonas Wintermans (R). Image courtesy of Additive Industries.
Highlands has acquired the shares of the startup’s CEO and co-founder, Daan Kersten, who will leave the company by June 30, 2020. In the interim, Chief Technology Officer Mark Vaes, who has been with Additive Industries since 2013, will fill the role. Kersten said of the decision:
“This substantial investment confirms the long-term commitment of Highlands to the growth ambitions of the company and it allows Additive Industries to make yet another significant step on its mission to revolutionize the productivity for the additive manufacturing of high-quality metal parts. After eight intense years of fast growth I feel the time is right to make way and hand over the reins to new leadership.”
The firm has quickly rolled out a modular metal PBF system with a high degree of automation and throughput. By reducing the need for operator intervention, the MetalFAB1 system is able to produce parts more rapidly, with pre- and post-processing operations happening in parallel to the build job. The next step in its roadmap was the development with SMS Group of automated factories called the Scale4Series, in which parts can be printed and post-processed automatically. In the process, Additive Industries has earned a number of high profile partners and clients, including Airbus/APWORKS, Volkswagen and the Sauber F1 team.
As Highlands is increasing its share of the 3D printing firm, it’s worthwhile to learn a bit more about the company. In fact, Highlands now says that it owns Additive Industries, in addition to a cigar machinery manufacturer, ATD Machinery, and NTS Group, which produces optomechatronic systems and mechanical modules for original equipment manufacturers. Interestingly, the CEO of NTS is also stepping down this August.
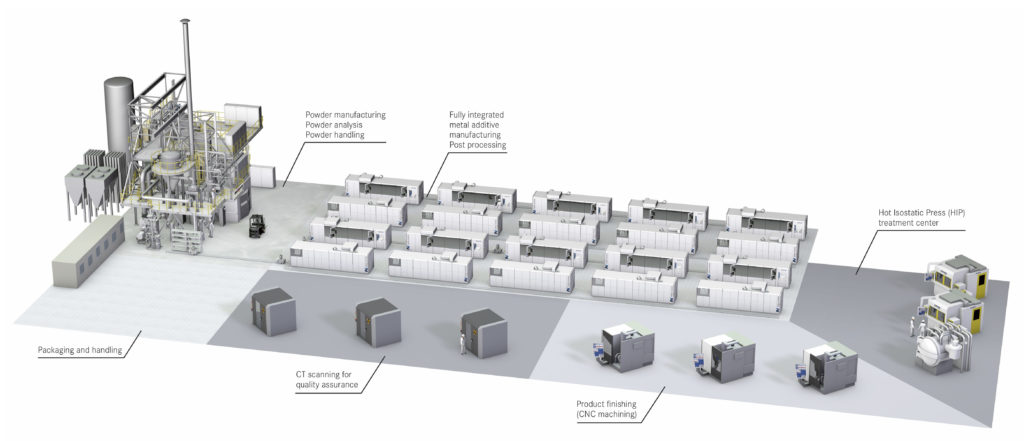
A rendering of the Scale4Series in development by Additive Industries and SMS Group. Image courtesy of Additive Industries.
Highlands is owned by the Wintermans, a Dutch family that founded and ran Royal Agio Cigars, one of the largest cigar manufacturing businesses in Europe, before selling it to Scandinavian Tobacco Group last year. The family divvied up 10 million Euros among its employees as a part of the deal. Highlands maintains its ATD business, meaning that it will continue to focus on the tech side of cigar making, but its investment in Additive Industries and its ownership of NTS Group signifies a continued shift in the family’s business operations overall, which previously had been making cigars since 1904. The sale of Royal Agio seems to suggest that the transition of Highlands from a cigar company to a tech company is near complete.
The post Additive Industries CEO Daan Kersten Steps Down as Firm Receives $14M Investment appeared first on 3DPrint.com | The Voice of 3D Printing / Additive Manufacturing.
4 Remarkable Creations in 3D Printed Furniture
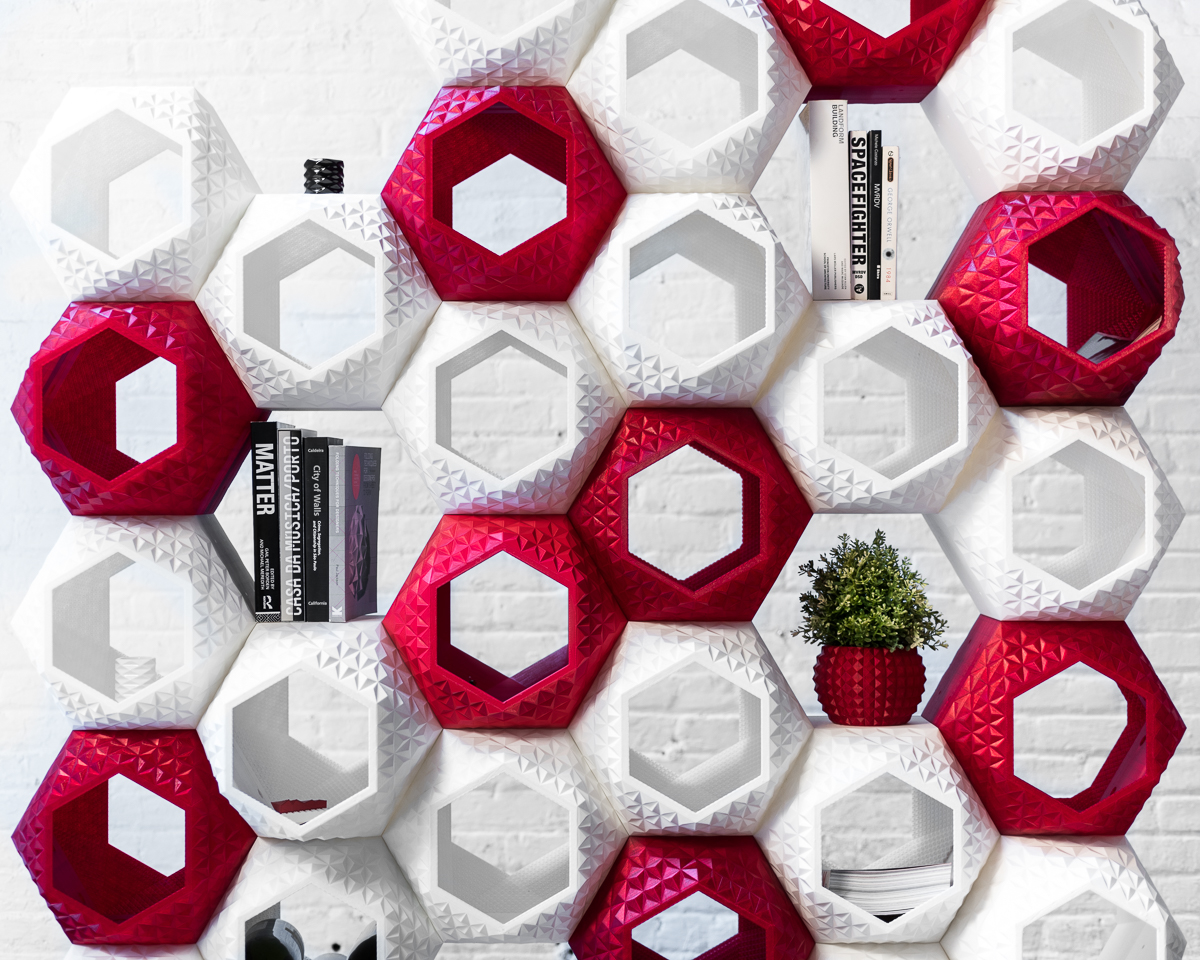
The use of 3D printing in furniture design has
allowed for the creation of highly precise, intricate and functional works of
art that use materials in fresh, imaginative ways. Many designers are using 3D printing
to create modern, sometimes futuristic furniture that uses the aesthetic of
machine made textures to its advantage. Here are a few to get started:
Folding Stool Printed by SLS Technology
In 2006, Patrick Jouin from Belgian design company Materialise.MGX created the ultimate practical folding seat with the One Shot Stool. The stool is designed to twist in one simple motion from a narrow folded position not much larger than a walking stick or folded umbrella into a sturdy and functional seat. The stool has a modern, fresh aesthetic and is easy to transport and store. The stool was printed using Selective Laser Sintering and the stool and all of its moving parts and hinges were cut out of the same piece of material.
The Most Intricately Detailed Chairs You’ve Ever Seen
Team CurVoxels from the Bartlett School of Architecture in London created a series of robot-printed filigree chairs using the designs of a S-curved chair as their basis. These highly intricate and complex chairs consist of thousands of tangled looking filaments in varying densities depending on where structural support needed to be strongest. The team created a custom nozzle for their printer that was able to produce four to six millimeter filaments in the air that allowed for uninterrupted printing and the students were able to alter the pattern where needed using an app.
A Versatile Modular Shelf for Spaces of All Sizes
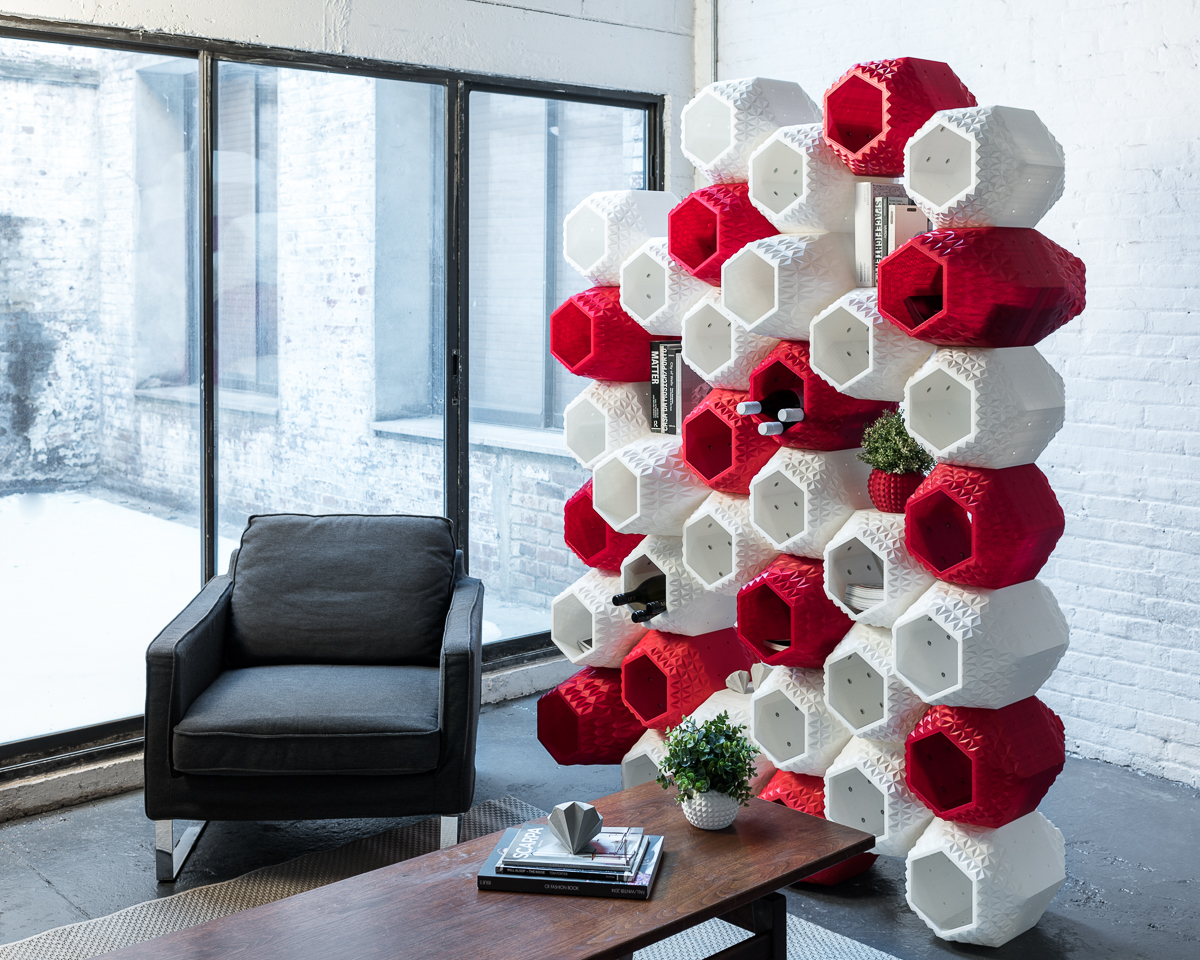
Sebastian Misiurek and Arianna Lebed designed SuperMod as a multi-faceted unit that could act simply as a shelving unit but also as a partition or standalone piece. Each of its mods were printed in opaque white and translucent red plastic and can be detached and moved around to suit specific storage needs as well as allow light to travel through it in different ways and create glowing effects. Using on-demand 3D printing, this versatile modular shelf can consist of as many individual units as one chooses to achieve the desired look or to fit a client’s specific storage needs.
Nature Inspired, Artificially Manufactured
The first collection produced by Spanish design company, Nagami Design, was a series of four 3D printed chairs, collectively named Brave New World, designed by Zaha Hadid Architects, Ross Lovegrove and Daniel Widrig. Bow and Rise, the first two chairs (Zaha Hadid), were inspired by coral reef and marine ecosystems and both feature a curved seat and a singular base printed by a pellet-extruder. The RoboticaTM stool (Ross Lovegrove) draws connections between natural programming and artificial manufacturing that occurs in robotics. The seat is made of silicone inserts and the seat itself was created using a continuous rotational process fusing layer upon layer together. David Wildrig’s Peeler chair is composed of three curved, seven-millimeter-thick shells of PLA plastic and was designed to use as little machine time and create as little waste as possible.
Handcrafting is still just as viable a way to create furniture, as that produces its own particular aesthetics. 3D printing simply allows for the further exploration of what aesthetics are possible and allows for there to be a focus on different types of details and methods, broadening the scope of what designers can create.
Feeling inspired? Let Shapeways 3D print your creation today.
The post 4 Remarkable Creations in 3D Printed Furniture appeared first on Shapeways Blog.
Greek researchers determine the effect of recycling on ABS filament
Texas A&M Researchers Make “NICE” Bioinks to Create Functional Bone Tissues
Managing bone defects and injuries using traditional treatments can be slow and expensive. When bones break, bone cells can usually repair them, unless the break is too large. In that case, clinicians have historically turned to bone grafts using non-essential segments of bone taken from other parts of the same patient; bone from the hips, pelvis, chin, or ribs can do the job. Unfortunately, this requires additional surgeries, which translates into more pain for patients, and basically, there is a limit to how much non-essential bone surgeons can take from a patient.
As in many fields, bioprinting is disrupting the way healthcare specialists think about solving problems. So with an estimated 500,000 annual bone grafting procedures in the United States and more than 2 million around the world, an efficient bone substitute could change millions of lives. In a search to fabricate patient-specific, implantable 3D constructs for regenerative medicine, scientists in the Department of Biomedical Engineering at Texas A&M University have developed a new bioink formulation for 3D bone bioprinting called NICE, which is short for Nanoengineered Ionic–Covalent Entanglement, and have gone on to demonstrate that this bioink can precisely reconstruct large bone structures based upon CT scans that were obtained from actual patients.
Led by Akhilesh K. Gaharwar, an associate professor in the Department of Biomedical Engineering, the research group developed a highly printable bioink as a platform to generate anatomical-scale functional tissues. Their study was recently published in the American Chemical Society’s Applied Materials and Interfaces scientific journal, whereby they state that the NICE bioinks allow precise control over printability, mechanical properties, and degradation characteristics, enabling custom 3D fabrication of mechanically resilient, cellularized structures.
Bioprinting requires cell-laden biomaterials that can flow through a nozzle like a liquid, but solidify almost as soon as they’re deposited. This is why bioinks need to act as both cell carriers and structural components, requiring them to be highly printable while providing a robust and cell‐friendly microenvironment. However, the research group realized that many current bioinks lack sufficient biocompatibility, printability, structural stability, and tissue‐specific functions needed to translate this technology to preclinical and clinal applications.
To address this issue, Gaharwar and his team are leading efforts in developing the more advanced NICE bioinks, essentially a combination of two reinforcement approaches, ionic-covalent entanglement, and nanoreinforcement. In fact, the researchers claim that to design the NICE reinforced bioinks for osteogenic tissue bioprinting, the bioink must be highly printable, mechanically strong, induce osteogenic differentiation, and be biodegradable. However, the difficulty of combining these requirements into a single bioink has been a major obstacle in bioprinting since its inception. So by combining these two distinct reinforcement methods, NICE becomes a robust and superior bioink while providing a highly hydrated and cell-friendly microenvironment for bone bioprinting.

NICE printed structures are highly flexible and resilient, as seen in these 3D printed tube structures that can be completely collapsed and quickly regain their shape (Credit: Texas A&M Engineering)
According to Texas A&M Today, Gaharwar said that developing replacement bone tissues could create exciting new treatments for patients suffering from arthritis, bone fractures, dental infections, and craniofacial defects.
“The next milestone in 3D bioprinting is the maturation of bioprinted constructs toward the generation of functional tissues,” Gaharwar said. “Our study demonstrates that NICE bioink developed in our lab can be used to engineer 3D-functional bone tissues.”
To illustrate the practical utility of NICE bioinks for bone tissue reconstruction, the team demonstrated how to create full-scale bioprinted implants customized for craniofacial defects on real patient CT scans. Relying entirely on open-source software, they used the free 3D modeling software Meshmixer to process the models and create bone defects, and the 3D printing applications PrusaSlicer and Repetier Host to bioprint the scaffolds. After bioprinting, the scaffold was crosslinked and implanted in a thermoplastic model of the lower jaw to demonstrate the closeness of fit. Strength of fit was also demonstrated by injecting and crosslinking NICE bioink between two sections of a full-thickness fracture to prove that NICE is able to quickly adhere surfaces together and resist shearing and delamination forces.
Funded by the National Institutes of Health (NIH)’s Director’s New Innovator Award, a National Science Foundation (NSF)’s Award and an X-Grant from Texas A&M University, the researchers suggest they have discovered a new way to design and produce 3D bioprinted bone tissue to benefit bone regeneration.
Moreover, Gaharwar claims to have demonstrated that the highly printable NICE bioinks can precisely reconstruct large bone structures from CT scans obtained from actual patients. The aim of the research is to enable patient-specific bioprinting of bone scaffolds to precisely match their injuries. The researchers stated their desire to have this technique act as a customizable and easy to work with an alternative to autografts that will provide surgeons with greater options for bone surgery. And with the ultimate goal of getting NICE bioink technology from bench to bedside, Gaharwar’s team plans to establish the in vivo functionality of the 3D bioprinted bone tissue.
The post Texas A&M Researchers Make “NICE” Bioinks to Create Functional Bone Tissues appeared first on 3DPrint.com | The Voice of 3D Printing / Additive Manufacturing.